The goal of the Research Grant and Fellowship Programs will fund critical research to increase understanding of the genetic and pathological processes involved in PKD and to accelerate the development of potential therapies for PKD patients.
The review process
The Review Committee was comprised of the PKDF Scientific Advisory Panel (SAP), additional ad hoc scientists and experts in PKD, as well as a Stakeholder Review Panel made up of PKD patients and caregivers. Each application was assigned three independent reviewers who ranked the grants based on our Guidance for Reviewers that provided separate criteria for scientists and stakeholders. Read more about our Peer Review Process here.
Rankings were based on:
- significance to PKD research
- innovation
- investigator strengths
- scientific environment
- approach
2024 research grant awardees
We are excited to share with you the ten grants and three fellowships selected for funding in 2024.
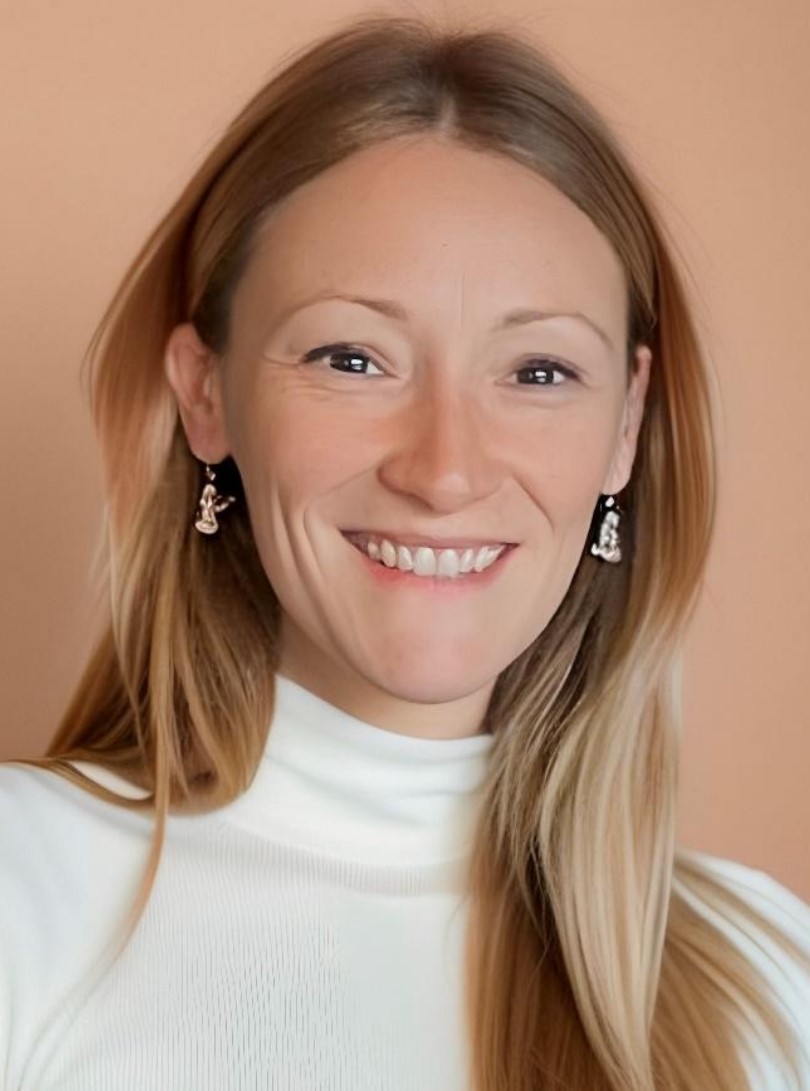
Vienna Brunt, Ph.D.
University of Colorado Anschutz Medical Campus
Project Summary
Cardiovascular and renal effects of mitochondrial-targeted antioxidant therapy in ADPKD
Autosomal dominant polycystic kidney disease (ADPKD) is the most common inherited kidney disease and accounts for 5-10% of cases of end-stage kidney disease worldwide. Importantly, because ADPKD involves a genetic mutation that affects tissues throughout the body, it is accompanied by abnormalities in organs besides the kidney, known as “extra-renal manifestations”. A major site of extra-renal manifestations of ADPKD is the cardiovascular (CV) system. Consequently, patients with ADPKD are at greatly elevated risk of CV diseases and events, including heart attacks, aneurysms, and sudden cardiac death. Some therapies are currently available to treat CV manifestations of ADPKD, including blood pressure medications. However, CV diseases remain the leading cause of death in ADPKD despite these treatments. Therefore, new therapies are needed.
CV manifestations of ADPKD include dysfunction of the arteries, high blood pressure (hypertension), and an impaired ability of the heart to pump blood efficiently. All of these can directly cause CV diseases. Of these, artery dysfunction shows up very early in the disease course, as early as childhood and before other CV manifestations are detectible. Artery dysfunction is also likely a cause of high blood pressure and heart problems in ADPKD. Therefore, therapies that target the arteries may be the most effective for reducing risk of CV diseases in ADPKD. In addition, artery dysfunction can cause damage to the kidneys and our preliminary data suggest that this could contribute to the growth of kidney cysts. Therefore, therapies that improve artery function could not only reduce CV risk in ADPKD but also have the potential to slow kidney disease progression, which could have a major impact on the lives of patients with ADPKD.
We use a mouse model of ADPKD that has one of the most common mutations in the Pkd1 gene found in human patients, the C57Bl/6J Pkd1RC/RC mouse. As a result, these mice develop cysts in their kidneys in a way that mimics the slowly progressing nature of ADPKD in humans. We have also found that these mice have many of the same abnormalities in the CV system as patients with ADPKD. In our preliminary studies using this mouse model, we have identified the dietary supplement MitoQ as promising new therapy to treat artery dysfunction in ADPKD. We have also seen that MitoQ can slow the growth of kidney cysts and that it may improve heart function. MitoQ is an antioxidant that targets the mitochondria, organelles in cells that use oxygen and fuel from food to produce energy. The mitochondria become dysfunctional in ADPKD and over produce free radicals that cause damage to cells, which we have shown is a key mechanism of artery dysfunction in ADPKD and which can be suppressed by MitoQ.
Therefore, the purpose of this PKD Foundation Grant is to perform a preclinical trial in our mouse model of ADPKD in which we will rigorously test the effectiveness of oral supplementation with MitoQ for improving artery function, slowing kidney cyst growth, and improving other CV manifestations of ADPKD (lowering blood pressure and improving heart function). Importantly, because artery dysfunction develops so early in the disease course but because patients are typically not diagnosed until later, we will compare the effectiveness of MitoQ between when it is initiated early vs. later in PKD progression. This will provide unique insight into the population of patients with ADPKD for whom MitoQ may be most beneficial. We will also perform various measures to identify the molecular mechanisms linking improvements in artery function to effects on the kidney, which could be relevant not only for understanding the effects of MitoQ but also for developing other therapies for slowing kidney disease progression. Importantly, studies that have dual emphasis on both the CV system and the kidney are rare in PKD research. Thus, our approach is highly innovative.
This will be the first study assessing the effectiveness of MitoQ supplementation in ADPKD. As such, a preclinical study in mice is needed first. However, we have designed our studies with translation to human patients in mind as the next step. For example, supplementing MitoQ to mice in their drinking water mimics giving a dietary supplement in humans. MitoQ is currently available as a dietary supplement in humans and has been shown to be safe with minimal side effects in initial clinical trials in humans in other disease populations. As such, our studies will provide strong support for rapid translation of MitoQ to young and adult patients with ADPKD as the next step.
Biography
Vienna Brunt, Ph.D., is an Assistant Professor in the Division of Renal Diseases and Hypertension at the University of Colorado Anschutz Medical Campus. She received her Ph.D. in Human Physiology from the University of Oregon and completed postdoctoral training at the University of Colorado Boulder in Integrative Physiology, with focus on cardiovascular physiology in both training experiences. Her research is aimed at developing novel interventions to improve cardiovascular health across the lifespan, with the goal of reducing morbidity and mortality associated with cardiovascular diseases. She recently expanded her interests to study cardiovascular dysfunction associated with kidney diseases, as cardiovascular disease is the leading cause of death in kidney disease patients. She is especially interested in better understanding and treating artery and heart dysfunction in autosomal dominant polycystic kidney disease. Her research approaches span the full translational spectrum, elucidating mechanisms and testing new interventions in cell/tissue culture and rodent models, and then translating promising interventions to initial clinical trials in patients.
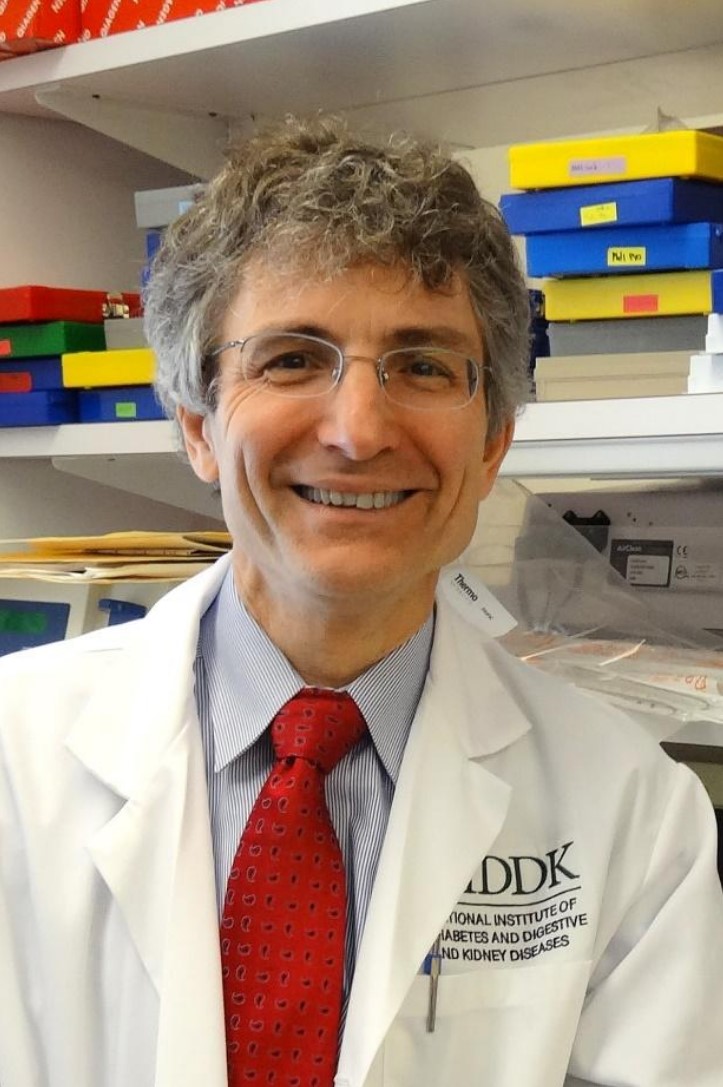
Gregory Germino, M.D.
National Institute of Diabetes and Digestive and Kidney Diseases, NIH NIDDK
Project Summary
Comprehensive analysis of the transition to the cystic state using a novel organoid system
ADPKD poses unique challenges for both those affected by the condition and those seeking to develop treatments. The disease is usually slowly progressive, taking decades before it causes the kidneys to fail, though this process can often be marked by episodes of recurrent pain, bleeding and/or infection. About half of those who have the disease progress to end stage kidney disease. Unfortunately, our ability to identify those at most risk of severe disease or rapid progression are limited, relying either on genotype (PKD1 truncating mutations are associated with most severe disease), phenotype (kidney volume adjusted for height, age, potentially other factors like hypertension), or some combination of these factors. None of these predictive algorithms are perfect, and those that rely on phenotype identify those who already have significant progressive disease. Ideally, one would like to intervene before such a stage.
PKD science has made considerable progress since identification of the causative genes, culminating in the first approved therapy for ADPKD. Despite these encouraging steps, the therapy is expensive, not well-tolerated by many, and at best offers modest benefit. The field clearly needs new therapies informed by the biology of the disease, but the challenge is in identifying treatments that can be given safely. Both the slowly progressive nature of the disease and the variability in its severity necessitate that treatments be both effective AND safe since they may need to be given for decades.
Therapeutic development could take multiple tracks:
a) correct the underlying genetic abnormality: The obvious solution for a genetic condition would be to correct the underlying genetic abnormality, and incredible developments in gene editing and gene delivery have certainly heralded a new age of genetic treatments. But the kidney poses real challenges for gene delivery and/or delivery of gene editing agents. Furthermore, separate treatment would likely be required for the liver, and we are not even sure what the appropriate target would be for vascular structures (ie. to prevent aneurysms).
b) correct the mutant gene or protein’s abnormality: Some mutations introduce a “stop codon” into the gene which causes the protein translation machinery to stop prematurely. Classes of drugs in development can override this feature, though their long-term efficacy and safety are unestablished with many uncertain about their likely value. Similarly, drugs have been developed for other conditions (eg cystic fibrosis (CF)) that can partially overcome the effects of mutations that reduce but do not eliminate a protein’s function. In the case of CF, investigators used a cell-based system to screen for efficacious agents. In ADPKD, this class of mutations is most often associated with milder disease, potentially limiting their value.
c) replace the mutant gene’s function or target some of its downstream pathways: Even if one can’t replace or correct mutant protein function, one might be able to replace its activity partially or in full if it is known. This approach requires a detailed understanding of PKD proteins and their effector pathways. This is an area where there are large gaps in our knowledge. Despite knowing for decades the major genes and proteins that result in ADPKD when disrupted, we still have a very incomplete understanding of the proteins’ normal functions. Importantly, we have even less of a consensus on how disruption of their function ultimately triggers cyst formation. These large gaps in our fundamental knowledge about normal function and pathobiology greatly hinder our ability to develop effective therapies for this condition.
d) target some of the aberrant signaling pathways driving disease: In this approach, one studies mutant cells and tissues to identify targets. While this may be helpful as treatments could slow disease progression or reduce some of its worst effects, they are not curative and often incompletely effective. Tolvaptan, which blocks the vasopressin-2 receptor, is an example. It provides very modest benefit, and it is not tolerated by all and has significant side effects.
Most of the strategies listed above would greatly benefit from three resources: a) a detailed knowledge of PKD protein function and downstream pathways; b) a detailed understanding of the steps between gene dysfunction and cyst formation and growth; c) a simple system for studying disease and testing or screening for therapies.
Our central hypothesis is that a simple, robust, cell-based system can be used to partially meet all three goals, and the project proposed in this application was developed to meet this need.
We have developed a simple, mouse-based cyst-forming organoid system that reliably (100%) develops cysts in <10 days. An important feature is that they also appear to be properly oriented, with primary cilia that face into the cyst lumen as occurs in vivo. This offers several distinct advantages. It allows very intensive interrogation of the signaling systems and pathways that go awry when Pkd genes are mutated since the process is very quick and predictable. Cyst formation is easily visualized since the organoid is in vitro, and the system can be easily and quickly manipulated to introduce reporters or modify downstream pathways. The system is scalable, and because the in vitro system is derived from mice, any findings can be relatively easily validated in an in vivo setting using the same mouse line. Finally, it is unique in that it is the first organoid system with correct ciliary orientation, a limitation of others that has been repeatedly raised in PKD RRC Conferences. In this application, we propose to use this system to deeply query the cellular phenotype during the very earliest stages of Pkd1 cyst formation using a variety of “omic” technologies and determine if the findings are shared by the Pkd2 cyst model, as would be predicted.
Biography
Dr. Germino is the Deputy Director of the National Institute of Diabetes and Digestive and Kidney Diseases (NIDDK) at the National Institutes of Health, an Adjunct Professor at Johns Hopkins School of Medicine, Acting Branch Chief of the Kidney Disease Branch of the NIDDK Intramural Research Program, and Section Chief of the Polycystic Kidney Disease Laboratory in NIDDK’s Division of Intramural Research. Dr. Germino received his undergraduate degree from Loyola University of Chicago, his medical degree from the Pritzker School of Medicine at the University of Chicago, trained in internal medicine and nephrology at Yale, spent a research year at Oxford University, and was full time faculty at Johns Hopkins from 1992-2009 where he directed its Polycystic Kidney Disease Center. His research program has been exclusively focused on ADPKD and ARPKD research for over 30-years, with his most recent work focused on using mouse, cell culture, and nephron organoid models to determine the function of PKD proteins and the pathobiology of cystic disease.
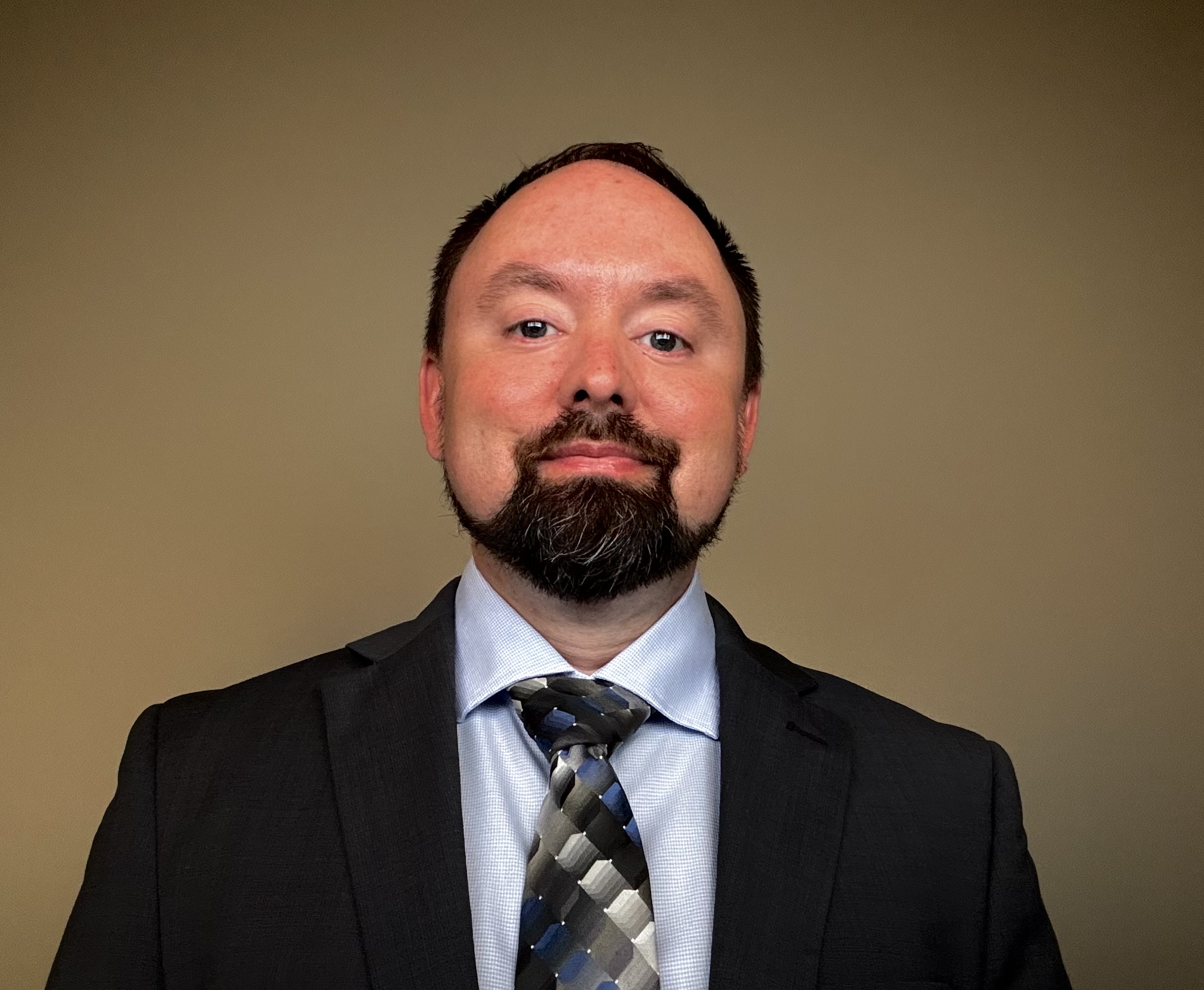
Timothy Kline, Ph.D.
Mayo Clinic, Rochester, MN
Project Summary
Developing a Digital Twin for Patients Affected by Polycystic Kidney Disease
Polycystic Kidney Disease (PKD) is a genetic condition characterized by the growth of numerous cysts in the kidneys, which can lead to serious health issues, including kidney failure. For those living with PKD, and their families, managing and understanding this disease poses a significant challenge. Traditional methods of tracking PKD have typically involved periodic medical assessments, which, while helpful, may not fully capture the ongoing changes in the disease. Our innovative project aims to bring a transformative solution to this problem, benefiting patients and stakeholders in the PKD community.
At the heart of our project is the development of a ‘digital twin’ – a groundbreaking concept in medical technology. Imagine a virtual model of a patient, so detailed and accurate that it mirrors the exact state of their disease in real-time. This digital twin is not just a static representation; it’s a dynamic model that evolves as the patient’s condition changes, providing continuous monitoring and offering insights into the disease’s progression.
The impact of our project on the PKD community is transformative. The digital twin marks a significant advancement in personalized medicine, shifting from traditional, periodic health assessments to a more continuous and comprehensive framework. Rather than being limited to snapshots in time, the digital twin provides a detailed, ongoing representation of a patient’s kidney health. This model allows for a deeper understanding of the disease’s progression over time, facilitating more informed and proactive treatment decisions. Such a nuanced approach is critical in managing PKD effectively, potentially delaying or preventing the progression to kidney failure, a significant concern for those affected by PKD.
For healthcare professionals, the digital twin offers an unprecedented tool for understanding and managing PKD. Armed with detailed data, doctors can tailor treatments to each patient’s specific needs, potentially improving outcomes and offering a more patient-centric approach to care. The predictive capabilities of the digital twin model also mean that clinicians can anticipate and prepare for changes in a patient’s condition, rather than reacting to them.
Beyond individual patient care, this project has broader implications for the PKD community. The data gathered from multiple digital twins can contribute to a richer understanding of PKD. This could lead to the discovery of new biomarkers, inform the development of novel therapies, and even provide insights into how different factors, such as genetics or lifestyle, affect the progression of PKD.
Importantly, our project also addresses disparities in healthcare. By providing a tool that can monitor and predict disease progression, the digital twin can help ensure that all PKD patients, regardless of their background or where they live, have access to the latest in disease management. This is especially crucial in PKD, where early intervention can make a significant difference in patient outcomes.
In summary, our project’s impact is far-reaching. It offers a new level of empowerment to patients, providing them with deeper insights into their condition and a more active role in their treatment. For clinicians, it brings a tool that enhances their ability to provide personalized care. And for the PKD community, it promises advances in understanding and treating this challenging disease. This is more than just a technological innovation; it’s a new horizon in PKD care, bringing hope and potentially transformative changes to the lives of those affected by the disease.
Biography
Dr. Timothy L. Kline is an Assistant Professor of Radiology at the Mayo Clinic, specializing in the application of artificial intelligence to medical imaging. His research focuses on developing advanced imaging techniques and AI algorithms to improve the diagnosis and management of patients affected by polycystic kidney disease (PKD). Dr. Kline’s innovative work aims to enhance patient care, contributing significantly to the fields of nephrology and radiology.
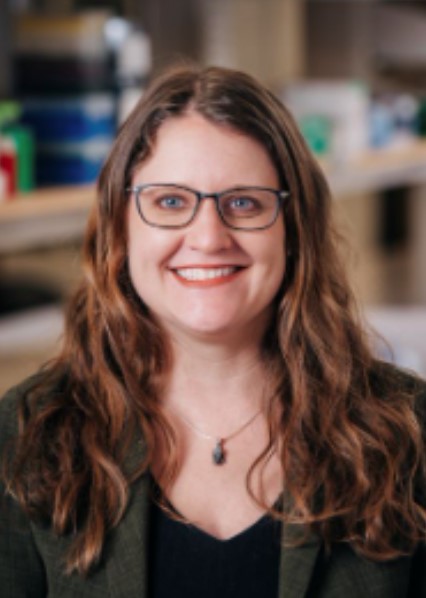
Brittany Lasseigne, Ph.D.
University of Alabama at Birmingham
Project Summary
Prioritizing drug repurposing candidates for PKD with machine learning and multimodal networks
Most autosomal dominant polycystic kidney disease (ADPKD) patients have both reduced quality and length of life (1). While tolvaptan was approved as the first and only ADPKD therapy for adults with a high risk of renal cystic disease progression (3). It reduces the rate of renal function decline by 30% (3-5), it does not cure ADPKD, shows no benefit for other PKD manifestations, is associated with liver toxicity, and costs ~$170,000 per patient per year (3). As new drug development typically requires years and billions of dollars, identifying drug repurposing candidates (new uses for already approved drugs) to stop, slow, or reverse ADPKD without severe adverse events is particularly promising. However, efforts to identify these drugs or drug combinations for ADPKD have largely been due to prior knowledge of perturbed pathways. They are further complicated by the challenge of modeling the complexity and high degree of interindividual heterogeneity in ADPKD. Despite advances in using preclinical models for human disease, most therapies still fail in phase III clinical trials (6).
Here, we propose to develop innovative machine learning and data science approaches for secondary data analyses utilizing existing ADPKD database resources. We will test the hypothesis that we can predict drug repurposing candidates or combinations of candidates targeting ADPKD kidney manifestations and prioritize those candidates for future testing by their predicted ability to succeed in both preclinical and clinical trials, as well as attributes known to be critical for clinical success (e.g., drug similarity, side effects). Our proposed research is innovative because it will 1) leverage cutting-edge machine learning and network approaches capable of detecting global patterns to identify drug targets, 2) identify drug combinations (shown to increase therapeutic efficacy while reducing toxicity in other diseases), and 3) prioritize drugs by predicted perturbation of both preclinical model and human kidney profiles while avoiding potential adverse events. The proposed research is significant because of the potential for identifying already approved drugs to bypass lengthy and costly drug development and because, by making version-controlled code to reproduce all analyses and prioritized drug candidates available as FAIR (Findable, Accessible, Interoperable, for Reuse) research products, we will enable the community to build upon our findings most effectively. This would impact ADPKD patient care more rapidly than developing novel drug targets and is responsive to the special consideration areas of innovative approaches to machine learning. As the Lasseigne Lab closely collaborates with the UAB PKD research community and Dr. Lasseigne serves as the Associate Director of the Center for Precision Animal Modeling Bioinformatics section, where her team leads efforts to identify drug targets and repurpose candidates for Mendelian diseases, they are uniquely positioned to execute the research proposed here, as well as follow-on proposals to evaluate prioritized repurposing candidates in preclinical models. While not the primary focus of this application, the computational approaches and prioritized drugs may also translate to other special consideration areas of interest to the PKD Foundation, including Autosomal Recessive PKD, ADPKD in children, lifestyle interventions (e.g., lifestyle mimetics), or extra-renal manifestations of PKD.
Biography
Brittany N. Lasseigne, PhD is an Assistant Professor in the Cell, Developmental and Integrative Biology department, an Associate Scientist in the Nephrology Research & Training Center, the Center for Neurodegeneration and Experimental Therapeutics, the Experimental Therapeutics section of the O’Neal Comprehensive Cancer Center, the Precision Medicine Institute, and the Informatics Institute at The University of Alabama at Birmingham (UAB) Heersink School of Medicine. Before joining UAB, Dr. Lasseigne trained in Biotechnology, Science, and Engineering at Mississippi State University (B.S.) and the University of Alabama in Huntsville (Ph.D.) and completed a postdoctoral fellowship in genetics and genomics at the HudsonAlpha Institute for Biotechnology. Her lab develops and applies genomic- and data-driven strategies to discover biological signatures that might be used to improve patient care and provide insight into the cellular and molecular processes contributing to disease, particularly polycystic kidney disease (PKD) and other diseases impacting the kidney. Their recent work includes prioritizing drug repurposing candidates for PKD, discovering sex-biased transcript expression, identifying sex-biased gene expression and regulatory patterns of drug targets and enzymes associated with sex-biased adverse events, and evaluating preclinical models and cross-species transcriptomic signatures for improving Mendelian disease research. The Lasseigne Lab is currently supported by funds from the National Institutes of Health and UAB and is focused on integrating genomics data, functional annotations, and patient information with machine learning and regulatory network approaches to discover novel mechanisms in disease etiology and progression, identify genome-driven therapeutic targets and opportunities for drug repositioning and repurposing, determine clinically relevant biomarkers, and understand how cellular context contributes to these diseases. By developing and applying single-cell, long-read sequencing, and advanced computational approaches to human diseases, they hope to accelerate future research. Dr. Lasseigne also serves as a program director and student research mentor for the NHGRI R25 Summer Undergraduate Research Experiences in Genomic Medicine (SURE-GM) program.
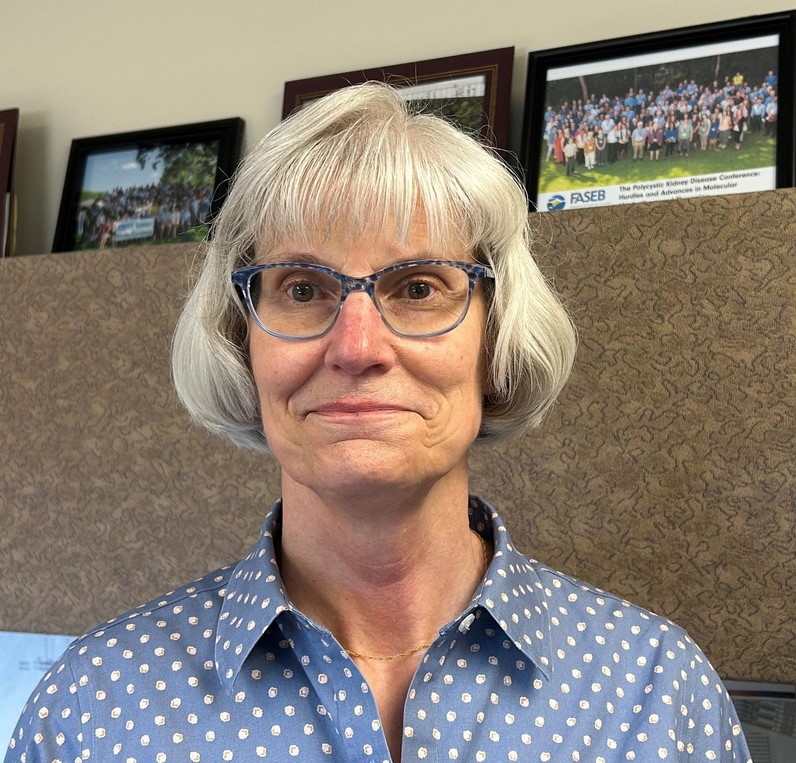
Robin Maser, Ph.D.
University of Kansas Medical Center
Project Summary
Development of targeted nanoparticle-based tethered agonist peptide mimetics for treatment of PKD
Autosomal dominant polycystic kidney disease (ADPKD) is the most common potentially lethal genetic disease. Our long-term goal is to develop safe and effective treatments for this disease. The predominant cause of ADPKD is mutation of the PKD1 or PKD2 genes, which encode the proteins polycystin-1 (PC1) and polycystin-2 (PC2). Together, PC1 and PC2 form a combined signaling receptor (PC1) and ion channel (PC2) complex within cellular membranes whose critical activity within the kidney is to maintain the normal structure and function of renal tubules. Importantly, PC1 and PC2 appear to be mutually dependent on each other for appropriate maturation, trafficking and functional activity or regulation. One of the leading ideas is that kidney cysts initiate when renal tubule cells have insufficient functional levels of this complex as a result of inherited and/or acquired mutations within their genes.
We know approximately 80% of the cases of ADPKD are due to mutations within the PKD1 gene, two-thirds of which result in a truncated and completely non-functional PC1 protein. However, up to one-third of the PKD1 gene mutations can produce PC1 protein but at reduced amounts (i.e., haploinsufficient) or with compromised function (i.e., hypomorphic) such that there are insufficient levels of PC1 activity to maintain a normal renal tubular structure. Excitingly, recent studies suggest that some PKD1 mutations may be amenable to therapies that increase the expression levels of PC1 protein. In a similar fashion, we believe that it is feasible to directly stimulate and augment residual PC1 activity as a treatment for ADPKD resulting from insufficient levels of either PC1 or PC2 protein. However, this type of therapeutic approach will not be possible without a better understanding of PC1 function(s) in preventing cystogenesis.
One of the proposed signaling functions of PC1 is as an unusual type of G protein-coupled receptor (GPCR). This is based on studies demonstrating that loss or alteration of PC1-mediated regulation of G protein signaling results in PKD in mouse and frog models. Unfortunately, we know very little about the mechanism by which PC1 functions as a signaling receptor or its immediate biological effects. We believe that understanding how PC1 functions as a signaling receptor will enable us to target and augment or regulate this activity as a treatment for ADPKD. We and others in the field have noted that PC1 shares multiple structural and functional features with the Adhesion family of GPCRs. Importantly, the mechanisms of signaling regulation have been uncovered for the Adhesion GPCRs, which provides clues for revealing PC1 function and its regulation. By using this analogy, we have investigated and shown that PC1 utilizes an Adhesion GPCR-like mechanism involving a tethered peptide agonist, or ‘TAP’ as we abbreviate it, which binds intramolecularly and activates the signaling function of PC1. The TAP is a short, ~20-amino acid sequence (i.e., peptide) located at the N-terminal end of the membrane-embedded portion of PC1, which can literally ‘bend backwards’ to interact with the remainder of the protein, resulting in activation of PC1 signaling.
In cell culture studies, we have now shown that synthetic peptides consisting of short stretches of the PC1 TAP sequence can be used to activate signaling (in trans) of PC1 that is missing its tethered agonist. Furthermore, we have found that treatment with the synthetic tethered agonist peptides (TAPs) can ameliorate cystogenesis (ex vivo) in embryonic kidney organ cultures from a mouse model with a hypomorphic Pkd1 mutation, thus supporting the physiological relevance of a PC1 tethered peptide agonist mechanism in cystic disease and the feasibility of activating it therapeutically. By partnering with a leading expert in nanotechnology, bioengineering and drug delivery, we will develop and characterize a nanoparticle-based therapeutic agent, i.e., ‘peptide nanosponges’ carrying our lead TAP. These ‘nanoTAPs’ will be synthesized with a covalently attached folate molecule to ensure their targeted delivery specifically to the kidney and cystic renal tubules. We will treat mice with a hypomorphic mutation of Pkd1 as a pre-clinical test of the therapeutic potential of our lead nanoTAP on cystic kidney disease. In the short term, successful completion of this project will demonstrate that nanosponges carrying a PC1 tethered agonist peptide can be specifically delivered to cystic kidneys and will provide ‘proof of concept’ that stimulation of PC1 signaling activity ameliorates cystic renal disease in vivo. Such results will also support the tethered agonist-mediated activation of PC1 signaling as a critical function and provide a strong foundation for the continued development of this novel therapeutic approach as a treatment for ADPKD which will be the focus of future research. Finally, if successful, this strategy is likely to be beneficial not only for ADPKD arising from PKD1, but also from PKD2 and other genes associated with cyst development in the liver and kidneys as a result of defects in the ER biogenesis of membrane proteins since these gene products reduce the level of PC1 protein and hence, PC1 activity.
Biography
Robin Maser, Ph.D., received her doctorate from the Department of Biochemistry and Molecular Biology at The University of Kansas Medical Center (KUMC) working on the transcription and function of small nuclear RNAs. She pursued postdoctoral studies under the direction of James Calvet, also at KUMC, which focused on identifying genes that were differentially expressed in cystic kidneys of the cpk mouse model of PKD. She later joined the faculty at KUMC and continued to work on multiple aspects of cystic kidney disease, including the signaling functions of polycystin-1, and the pathogenic mechanisms and treatment of ADPKD in collaboration with James Calvet, Jared Grantham, and Vince Gattone, respectively. Research from her lab demonstrated the membrane-embedded structure and biogenesis of polycystin-1. The current project is focused on testing the efficacy of a novel nanosponge-delivered agonistic peptide to stimulate the residual function of polycystin-1 in mouse models with reduced polycystins. When not working in the lab, Robin enjoys kayaking, pickleball, woodworking, and spending time with her furry ‘kid’ (a chocolate labrador retriever, Kodi).
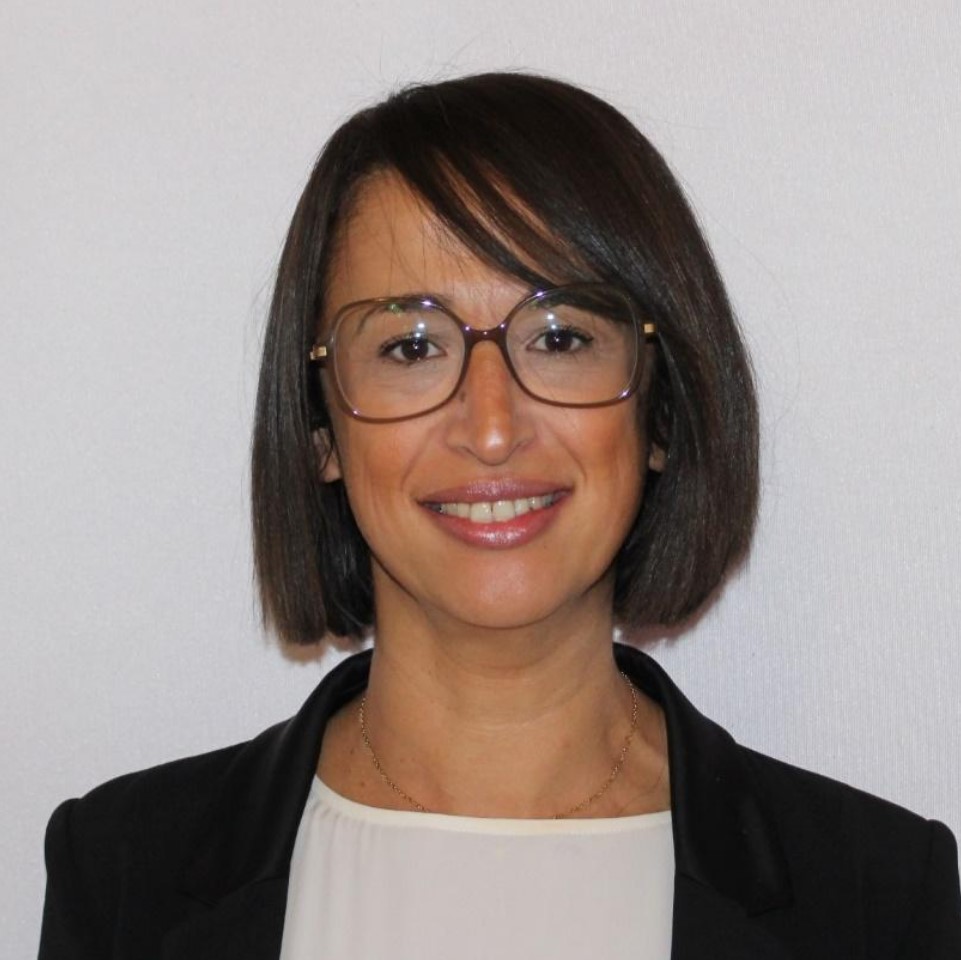
Djalila Mekahli, M.D., Ph.D.
University Hospitals Leuven (UZ Leuven)
Project Summary
Early biomarkers for stratification of disease progression in ADPKD children
In the previous years, our group has contributed to challenging the paradigm for the management of Autosomal Dominant Polycystic Kidney Disease (ADPKD) by demonstrating that this disorder manifests itself already in childhood. Spotlighting this simple but critical insight implies that in order to prevent progression of the disease to kidney failure (KF), treatment of ADPKD must start early, before a point of no return has been reached. For this purpose, new targets for safe and efficacious drugs need to be identified, but also novel biomarkers of disease progression in young ADPKD patients with early disease stages. Applying these biomarkers to a comprehensive classification system including imaging and clinical data for pediatric ADPKD patients would mean a significant breakthrough in the early ADPKD field and will lead to new insights into predicting outcome, designing trials and selecting the patients who are most eligible for therapeutic interventions for early stage ADPKD disease. By making this classification tool accessible to clinicians worldwide and easily applicable, it will have an essential impact on its management and will help in counseling families. In addition, the development of this classification system will shed light on the clinical phenotype of early ADPKD. This knowledge is essential to identify the best timepoint for intervention in the disease, and to delineate early events in disease progression.
Finally, by identifying known and novel biomarkers in the early stages of the disease, it will contribute to the identification of novel underlying players in the proximal events in cystogenesis, and potentially form a basis for novel drug target discovery in early ADPKD.
Biography
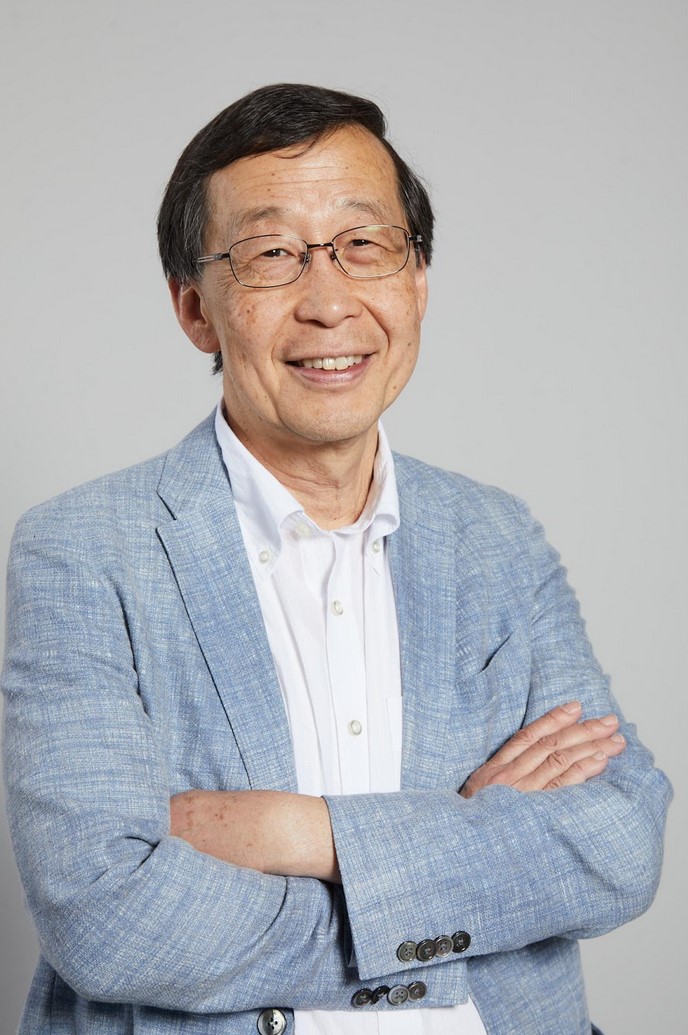
Albert Ong, M.D., BMBCh, MA, FRCP
University of Sheffield
Project Summary
Long non-coding RNA in urinary extracellular vesicles as early disease biomarkers in ADPKD
The course of ADPKD can vary considerably between individuals even within the same family and there is a clinical need to identify those with more rapidly progressive disease who would benefit from early treatment (eg tolvaptan) or more intensive management. Extracellular vesicles are tiny microscopic particles that actively exported by most cells in the body including the kidney. They may be a common way through which cells communicate with each other since they contain both protein and genetic messengers including small ribonucleic acid (RNA) molecules. By isolating these particles in urine, we could potentially ‘sample’ the kidney without the need to do a needle biopsy (‘liquid biopsy’). In this study, we will examine whether the RNA content of urine vesicles could be used to more accurately predict kidney lifespan, decide treatment options and provide clues for developing novel drugs.
Biography
Albert Ong is Professor of Renal Medicine at the University of Sheffield, UK. Born in Malaysia, he was educated at the University of Oxford, trained as a clinician-scientist at University College London and Oxford before taking up his present post. Work in his laboratory has focussed on understanding the molecular basis of cyst formation, the determinants of biological variation and drug discovery in ADPKD for which he received the 2022 ISN Lillian Jean Kaplan International Prize. He is Co-Director of the MRC-NIHR UK Renal Ciliopathies National Network (CILIAREN) and leads specialist services for patients with inherited kidney diseases at the Sheffield Kidney Institute. He has published over 200 papers, trained over 30 postgraduate students and fellows, lectured globally, provided strategic leadership and given expert testimony in the ADPKD field. He is a member of the ERA Council and served as Scientific Program Chair for the 61st ERA Congress in 2024.
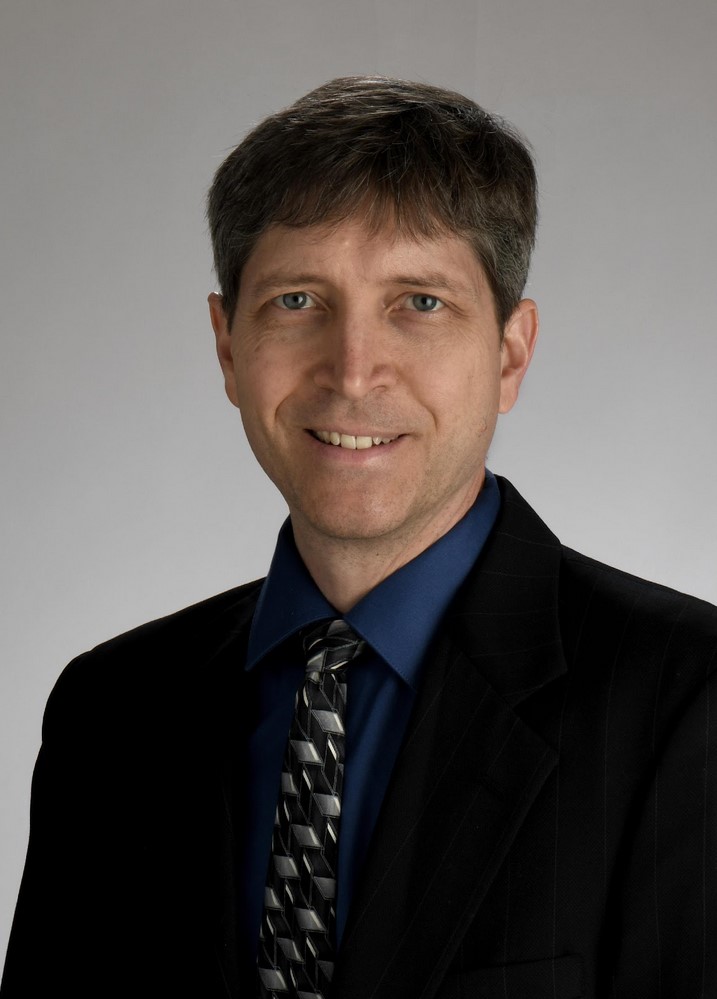
Stephen Parnell, Ph.D.
University of Kansas Medical Center
Project Summary
Effect of Pregnancy and Lactation on PKD
Autosomal dominant polycystic kidney disease is a common genetic disorder affecting 1 in 500-1000 individuals. Although the disease is inherited genetically, progression is variable within the patient population and is likely affected by diverse factors including diet, lifestyle, and epigenetic factors.
We have generated a mouse model with a slowly progressive form of cystic disease that mimics ADPKD. In our experience with this model we have obtained preliminary evidence that suggests that pregnancy exacerbates PKD progression. There is good evidence that the rate of PKD progression is different between males and females, but there has surprisingly been no investigations into pregnancy as a driver of cystic disease. The availability of this new mouse model allows the opportunity to study this possibility in a controlled environment. Furthermore, the increasing volume of clinical patient data from long-term imaging studies, such as CRISP, provide the opportunity to retrospectively address this question in human patients as well.
This proposal will merge basic science and clinical data-mining approaches to determine whether pregnancy is a risk factor for ADPKD patients. The information gained from this study will hopefully help patients make important lifestyle decisions with regard to their choices related to pregnancy.
Biography
Dr. Stephen Parnell, PhD, is a Research Associate Professor in the Department of Biochemistry and Molecular Biology, and a member of the Jared Grantham Kidney Institute at the University of Kansas Medical Center. His specific research interests are in polycystin-1 structure/function and aberrant cell signaling mechanisms that contribute to cystic disease progression. He has enjoyed working with many friends and colleagues in the area of PKD research since his introduction to the field in the mid 90’s, but was familiar with the disease for many years prior on account of having a parent with ADPKD. Dr. Parnell is deeply appreciative of the Foundation’s commitment to patient advocacy and financial support for research. Outside of the lab, Dr. Parnell enjoys hiking in the mountains (and other outdoor locations) and getting new summit photos in his ENDPKD shirt.
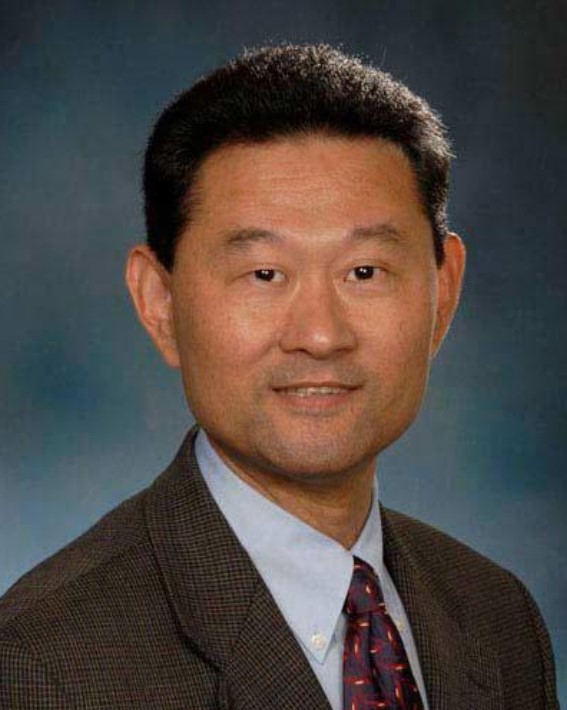
Feng Qian, Ph.D.
University of Maryland, Baltimore
Project Summary
Mitochondrial Role of Fibrocystin/Polyductin in Cyst Formation Through Its Cleavage Product ICD15
Fibrocystin/Polyductin (FPC) is the protein encoded by PKHD1, the principal gene responsible for autosomal recessive polycystic kidney disease (ARPKD). ARPKD is a severe neonatal nephropathy characterized by cystic dilation of collecting ducts and congenital hepatic fibrosis. Despite its significance, the exact function of FPC and its role in cyst formation remain elusive, posing challenges to the development of effective therapeutic strategies for ARPKD. Unexpectedly, Pkhd1 mutant mice exhibit only minimal renal disease, further restricting the study of FPC in ARPKD pathogenesis.
We recently published a study unveiling a crucial connection between FPC and mitochondria in cyst formation (Walker et al. 2023). This connection is likely mediated by proteolytic cleavage of FPC, releasing a novel intracellular C-terminal domain product known as ICD15. Our findings indicate that ICD15 enters the mitochondrion—the cellular organelle responsible for producing ATP for cellular energy currency and producing small molecular building blocks for larger molecules. Pkhd1 knockout (KO) mice exhibit structural and functional abnormalities in the mitochondria of kidney tubules. Moreover, Pkhd1 KO greatly worsens PKD in the Pkd1 mouse mutant known as Pkd1V/V. These digenic mouse mutants mimic ARPKD, particularly those severely affected due to biallelic mutations in PKHD1 with coinheritance of changes in an ADPKD gene. Furthermore, removing the ICD15 sequence from FPC aggravates PKD in Pkd1V/V mice. Our findings indicate that dysfunction of ICD15 could be a key factor in cyst growth
Our discoveries offer intriguing insights. First, the identification of mitochondrial abnormalities in Pkhd1 KO kidney tubules, even in the absence of cyst formation, rules out the possibility of them being a consequence of cyst formation. Instead, they likely result directly from FPC inactivation, establishing a pro-cystic state that operates synergistically with the Pkd1-dependent cystic pathway to promote cyst development. Second, the absence of ICD15 alone is the likely cause of mitochondrial dysfunction contributing to this pro-cystic state. The importance of ICD15 in pathogenesis is underscored by the identification of various mutations in PKHD1’s final exon (exon 67, encoding ICD15) in severely affected ARPKD patients. Third, ICD15, therefore, may normally play a crucial role in regulating mitochondrial function, potentially facilitating tubular adaptation in response to environmental cues. Fourth, our digenic mouse mutants mimicking ARPKD provide a valuable model for investigating FPC function in cystogenesis.
We hypothesize that ICD15 of FPC participates in a mitochondrial signaling pathway that is linked to cystogenesis operating synergistically with the Pkd1-dependent cystic pathway. Consequently, introducing an extra copy of ICD15 may alleviate cystic development in PKD. This concept addresses a knowledge gap in comprehending FPC function and its involvement in ARPKD. Validation of the hypothesis will likely provide novel insights into FPC’s function and its role in cyst growth through its ICD15 product and open new avenues for novel ICD15-based therapeutic interventions in ARPKD. Accordingly, our proposed research is designed with the following two main aims.
1. Elucidate the role of FPC in mitochondrial signaling via ICD15. We hypothesize that FPC-ICD15 interacts with other mitochondrial regulatory proteins and affects mitochondrial metabolism. Recognizing oxidative stress as a key contributor to ADPKD pathogenesis, we will determine the effect of Pkhd1 inactivation on the levels of reactive oxygen species (ROS), oxidative stress, and antioxidant defenses in the kidneys and cells, and assess if ICD15 expression may alleviate these effects. Furthermore, we will investigate the ICD15 signaling pathways associated with the disease through an unbiased approach, employing mitochondrial proteome and protein network analysis.
2. Assess suppressive effects and mechanism of ICD15 on renal cyst growth in Pkd1V/V mice. This will involve the development of a new transgenic mouse line expressing ICD15 and testing its effect in mitigating the cystic phenotype of Pkd1V/V mice. Additionally, we will use the MITO-Tag mice to facilitate mitochondria isolation from their kidneys. The isolated mitochondria will be subjected to mitochondrial proteomic and bioinformatic analyses to gain novel insights into the cyst-suppressive mechanisms of ICD15.
The anticipated results may yield significant impacts.
THE SHORT-TERM/IMMEDIATE IMPACT. This study may advance our understanding in several key areas:
• Defining the role of FPC and its ICD15 in regulating ROS and oxidative stress.
• Identifying the effect of FPC and the role of ICD15 on changing the mitochondrial proteome.
• Determining ICD15 binding partners in mitochondria and its protein interaction network
• Characterizing the ICD15 signaling pathway and the contributors to the pro-cystic state.
• Developing a transgenic mouse line expressing ICD15.
• Assessing whether transgenic ICD15 mitigates the cystic phenotype of Pkd1V/V mice.
• Delineating cyst-suppressive mechanisms of ICD15 through mitochondrial proteome analysis and functional annotation.
THE LONG-TERM IMPACT. The insights gained may pave the way for the development of interventions and therapeutic strategies based on ICD15 expression to mitigate cyst growth. Our findings may establish a foundation for innovative interventions, including small molecules, peptides, and nanobodies, designed to enhance the release of ICD15 from full-length FPC. Alternatively, small molecules that can enhance the function of the mitochondrial electron transport chain may be effective in compensating for the loss of ICD15. These FPC-directed therapeutic strategies hold considerable promise compared to previous attempted approaches that primarily focused on targeting altered signaling pathways associated with the disease.
Biography
I am a Professor of Medicine specializing in Polycystic Kidney Disease (PKD) research with a particular focus on the intricate biology of PKD proteins, including Polycystin-1 (PC-1), Polycystin-2 (PC-2), and fibrocystin/polyductin (FPC). With a robust foundation in molecular biology, biochemistry, animal models, and human PKD, I have firmly established myself as a principal investigator or co-investigator on numerous grants, generously funded by prestigious organizations including the NIH/NIDDK and various foundations including the PKD Foundation. Over the span of 25 years, my contributions to the PKD field have been profoundly impactful. A defining achievement was my pivotal role in sequencing the PKD1 genomic locus, a foundational step that underpinned the establishment of the two-hit model of cystogenesis. Moreover, I spearheaded the discovery of the interaction between PC-1 and PC-2, specifically elucidating their interactions through their C-termini. My laboratory’s findings have highlighted the critical role of PC-1’s autoproteolytic cleavage at its GPCR Proteolysis Site (GPS) in controlling the protein’s biogenesis, ciliary trafficking, and signaling properties. These accomplishments collectively provide me with an extensive grasp of polycystin protein biochemistry. In addition to my research efforts, I also serve as the director of the Molecular Core of the NIH U54-funded Polycystic Kidney Disease Research Resource Consortium. This pivotal role entails the development and dissemination of various PKD antibodies, alongside a rich array of reagents and expertise, contributing valuably to the expansive research community. Of particular note is the development of a distinguished collection of FPC-specific antibodies, as well as various FPC expression constructs.
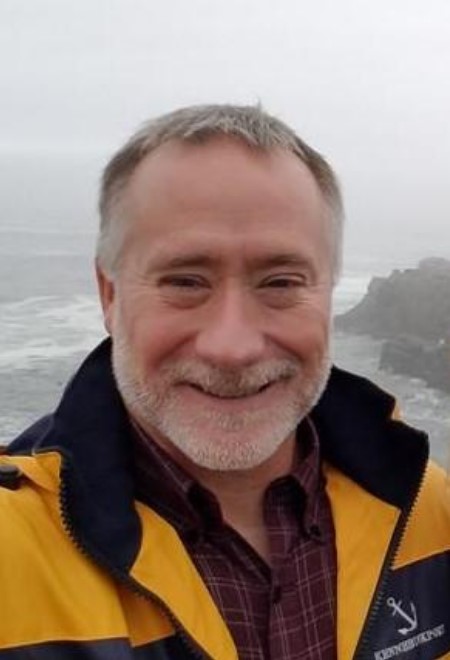
Darren Wallace, Ph.D.
Project Summary
Role of BRAF on renal cyst formation in PKD
Autosomal dominant polycystic kidney disease (ADPKD) is an inherited disorder with a diagnosed prevalence of 4.3 per 10,000 individuals affecting ~144,000 people in the US. The disease is characterized by the relentless growth of numerous fluid-filled cysts causing kidney injury, tissue inflammation, fibrosis (scar tissue), and progressive decline in kidney function. Approximately, one-half of ADPKD patients reach end-stage kidney disease by the 6th decade of life, accounting for 6-10% of patients on renal replacement therapy. Over the past two decades, there have been major advancements in PKD research; however, the lack of understanding of cellular pathways involved in initial cyst formation has limited our ability to design and develop effective therapies to prevent or halt renal cystic disease in ADPKD patients. Tolvaptan (Jynarque) is an important first therapeutic for the treatment of ADPKD; however, its side-effects, including polyurea (excessive urination), nocturia (excess urination at night), polydipsia (excessive thirst), and liver complications, limit its use in some patients.
Our research has shown that BRAF, a kinase upstream of the ERK pathway, is the key intermediate in the proliferation of human ADPKD cells. BRAF is normally inhibited or repressed in the kidneys. Mutations genes that cause ADPKD lead to de-repression of BRAF in the cystic cells such that hormones such as vasopressin, an antidiuretic hormone that works through a cell signaling molecule cAMP, stimulates BRAF and ERK-dependent cell proliferation. Recently, we generated a novel mouse that expresses a constitutively active BRAFV600E, a common activating mutation found in cancer, in the renal collecting ducts (CD)s of otherwise normal mice. These BRAFCD mice formed cysts in the CDs, which is a prominent site for cyst formation in human ADPKD. It is well established that cysts can form in all segments of the nephron; therefore, we will express active BRAF in all nephron segments of normal kidneys and identify which segments form cysts due to BRAF activation. In a preliminary experiment, we found that changes in gene expression during initial cyst formation in BRAFCD kidneys were similar to those activated during initial cyst formation in PKD mice, suggesting that the same pathways are activated in both models. Pathways analysis indicated that the top pathways affected were tissue remodeling and inflammation, which are also affected in human ADPKD kidneys.
Our hypothesis is that BRAF activation is sufficient and required for the initiation of cyst formation in ADPKD and that targeting BRAF prevents the initiation of cyst formation and halts disease progression in PKD mice.
In Aim 1, we will determine if the expression of active BRAF induces in vitro cyst formation of human kidney cells, and if active BRAF induces cyst formation throughout the nephron causing early inflammation of the kidneys. We will express an inducible BRAFV600E in primary normal human kidney (NHK) cells to determine if active BRAF drives ERK-dependent cell proliferation and in vitro cyst formation of cells seeded within a collagen matrix. Using the BRAFV600E mouse, we will express active BRAF throughout the entire nephron of wildtype mice, identify the nephron segments that form cysts, and evaluate changes in markers of cell proliferation, inflammation, fibrosis, and renal function.
In Aim 2, we will use novel gene editing approaches to knockout BRAF in human ADPKD cells to determine if the loss of BRAF prevents cell proliferation and in vitro cyst formation. A conditional BRAF knockout mouse will be used to determine if the loss of BRAF in the nephrons of Pkd1RC/RC mice prevents renal cyst formation and inflammation, and reverses gene expression and cellular pathways that were identified to be activated during initial cyst formation.
We think that these studies are important to the PKD field to understand initial cyst formation and activation of pathways involved in tissue remodeling and inflammation, and to the PKD patients. FDA-approved Raf inhibitors show efficacy on inhibition of the BRAFV600E protein in metastatic melanoma; however, resistance develops, in part, due to dimerization of RAF proteins making the drugs ineffective. There is growing interest in the development of next-generation RAF inhibitors that target both monomers and dimers of the RAF proteins. We think that a better understanding of the role of BRAF on the initiation of renal cystic disease in ADPKD is timely with the emergence of new BRAF inhibitors.
Biography
Darren Wallace is a Professor in the Department of Internal Medicine and a member of the Jared Grantham Kidney Institute at the University of Kansas Medical Center. Dr. Wallace earned his PhD from the Department of Molecular and Integrative Physiology and completed postdoctoral training in renal physiology and polycystic kidney disease (PKD) at the University of Kansas Medical Center. His contributions to the PKD field include research on the molecular mechanisms for cAMP-dependent cell proliferation and Cl–dependent fluid secretion using primary cultures of human PKD cyst epithelial cells. His laboratory discovered that periostin, a matricellular protein, is highly overexpressed by cyst-lining cells of PKD kidneys, where it binds to integrins and stimulates tissue repair pathways, leading to aberrant cell proliferation and matrix production, driving progressive cyst growth and fibrosis. Recently, his research has focused on the pivotal role of BRAF, a kinase upstream of the mitogen-activated protein kinase ERK, on cyst initiation in PKD. Dr. Wallace is the Director of the Kansas PKD Research and Translational Core Center and Director of PKD Biomarkers, Biomaterials and Cellular Models Core, which are part of the national PKD Research Resource Consortium (PKD-RRC). He also served as a member of the PKD Foundation Scientific Advisory Committee from 2008 to 2019.
2024 fellowships
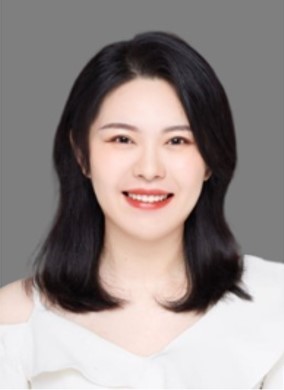
Xinyue Mao, Ph.D.
Mayo Clinic, Rochester, MN
Project Summary
Understanding mechanisms of senescence in promoting cyst growth in ADPKD
The roles of cellular senescence in ADPKD have become the focus of scientific investigation. However, whether senescent cells can directly drive ADPKD-related pathology and be therapeutically targeted is still unclear. To address these fundamental questions, we investigate whether senescence plays a fundamental and mechanistic role in the pathophysiology of ADPKD, identify key SASPs secreted by senescent Pkd1 null renal epithelial cells, and investigate the role of one candidate SASP in the regulation of cyst growth in ADPKD mouse models. Completion of this project will provide strong pre-clinical mechanistic data supporting the role of senescence in the pathophysiology of ADPKD and identify novel therapeutics that target fundamental senescence processes to improve both immediate and long-term outcomes related to ADPKD. Thus, this study is highly significance and therapeutically relevant with exciting potential for translation.
Biography
Xinyue Mao, MD, PhD. is a postdoctoral fellow under the mentorship of Dr. Xiaogang Li in the Division of Nephrology and Hypertension at Mayo Clinic. Her long-term career goal is to be a physician scientist in Nephrology. Her research projects focus on understanding molecular mechanisms of cellular senescence and epigenetics in autosomal dominant polycystic kidney disease (ADPKD) and discovering novel therapeutic strategies for ADPKD treatment. This PKD Foundation fellowship investigates how cellular senescence promotes cyst growth and test for the first time of whether removing of senescent cells delays cyst growth in ADPKD. In addition, this project addresses how senescent Pkd1 mutant renal epithelial cells regulate neighboring cell biology and function through the release of the novel identified senescence-associated secretory phenotype (SASP), such as AKR1B1. Completion of this project should facilitate a comprehensive understanding about the role of senescence in the pathophysiology of ADPKD and the development of novel therapeutic strategies that target the fundamental process of senescence to improve the outcomes related to ADPKD. Thus, this study is highly significant and therapeutically relevant with exciting potential for translation.
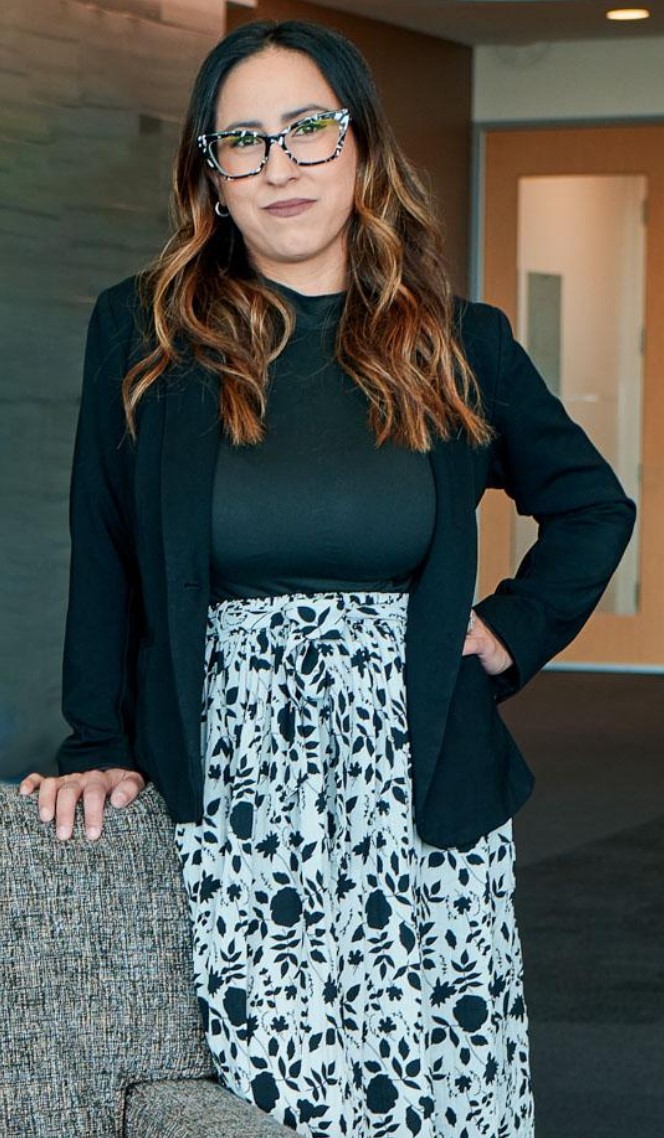
Karla Marquez-Nogueras, Ph.D., M.S.
Loyola University of Chicago
Project Summary
The PC2-dependent activation and regulation of the Aquaporin 2 signaling pathway
The kidney represents a unique environment where cells are exposed to a variety of different stimuli. Cellular homeostasis is critical for kidney cells as dysregulation of any signaling pathway can trigger the progression of kidney disease. The ability of renal cells to adapt to changes in their environment is critical for cell survival. Autosomal Dominant Polycystic Kidney Disease is the leading genetic cause of renal failure affecting ~1:1,000 people and has no cure. ADPKD is caused by loss-of-function mutations to PC2 where it causes the formation of renal cysts being the most damaging one in the collecting duct (CD) of the nephron where urine concentration is finalized. I have shown in my pre-published manuscript that deletion of PC2 in the CD leads to the activation of hyperosmotic dependent signaling pathways like the incorporation of Aquaporin 2 (AQP2) in the apical membrane of CD cells. However, it has not been previously characterized how do the polycystin proteins regulate and activate the osmotically induced AQP2 signaling pathway. This proposal aims to characterize how PC2 activates and regulates the osmotically induced AQP2 signaling pathway. The characterization of this signaling pathway provides an early intervention point for ADPKD patients and new signaling pathways that can be targeted for therapeutic development.
Biography
Dr. Márquez-Nogueras is a postdoctoral researcher in Dr. Ivana Kuo’s laboratory in the Department of Cell and Molecular Physiology at Loyola University Chicago. She earned her bachelor’s degree in Industrial Microbiology and her master’s degree in Microbiology from the University of Puerto Rico, Mayagüez and received her PhD in Microbiology from the University of Georgia. Dr. Márquez Nogueras’s research combines the use of molecular approaches, high-resolution live cell imaging, cell culture and animal models to undestand how breakdown of cellular communication in the PC2 signaling pathway can lead to defects in urine concentration. In the proposed study, Dr. Márquez-Nogueras will aim to characterize how PC2 activates and regulates the osmotically induced AQP2 signaling pathway.
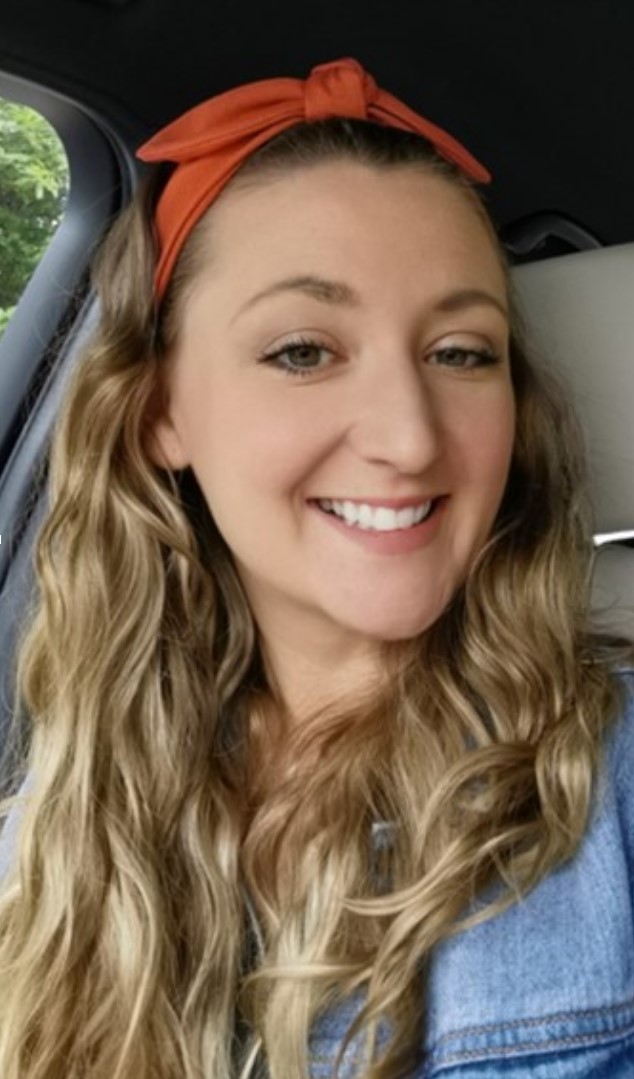
Marie-Louise Monaghan, Ph.D., MRes
University of Colorado-Denver
Project Summary
The role of adipose tryptophan metabolism in obesity-driven PKD progression
Autosomal dominant polycystic kidney disease (ADPKD) is characterized by growth of fluid-filled cysts in the kidney, resulting in loss of kidney function and kidney failure. To date, Jynarque is the only approved therapy that slows kidney cyst growth progression in patients with ADPKD. However, it is only approved for a subset of patients and lowers quality of life; as such, understanding mechanisms that drive PKD and finding new treatment options is vital. Obesity is a growing public health problem of which the ADPKD population is not spared. Work from our group has shown that, in patients with ADPKD, being overweight or obese increases the rate at which kidney disease progresses. In my preliminary studies, I developed an overweight mouse model with ADPKD that presents with more rapid disease progression compared to normal weight mice, mimicking human findings. As it is difficult to acquire kidney, blood, and adipose tissue from patients with ADPKD, this model uniquely allows us to decipher mechanisms that link adiposity and rapid kidney cyst growth. Importantly, overweight/obesity results in the global dysregulation of multiple signaling pathways, including metabolism and organ inflammation, which have been shown to influence kidney cyst growth in mouse models of ADPKD. This includes metabolism of the amino acid tryptophan (TRP). I have recently published a paper linking tryptophan, its metabolizing enzyme IDO1, and its metabolites kynurenines to the progression of ADPKD in normal weight mice. Of significance, in overweight non-diseased mice, I found increased expression of IDO1 on adipose immune cells (macrophages) and increased levels of kidney kynurenine compared to normal weight mice. Plasma collected from patients with ADPKD and overweight/obesity shows that kynurenine levels rise with increasing body mass index. In this application, I hope to achieve a detailed characterization of adipose TRP metabolism as overweight/obesity progresses with a focus on understanding which cells within this secretory organ are critical to the dysregulation of the pathway, as well as its impact on systemic/kidney inflammation and subsequent kidney cyst growth. I will also test whether returning from an obese to a lean state resolves dysregulated TRP metabolism and slows kidney cyst growth. To increase the translational potential of my studies I will assess if IDO1 loss in adipose macrophages slows adipose-driven kidney cyst growth and whether blocking IDO1 with an FDA approved inhibitor corrects the pathogenic function of these macrophages in cell culture. These findings will provide foundational and mechanistic data that allow testing of an FDA approved inhibitor of TRP metabolism as a therapeutic option for patients with overweight/obesity and ADPKD. These findings could augment the progression of this common, detrimental, and economically costly diseases as well as inhibit its obesity-associated accentuation.
Biography
Dr. Marie-Louise Monaghan Ph.D. is a Postdoctoral Research Fellow within the lab of Dr. Katharina Hopp at the University of Colorado Anschutz Medical Campus, Department of Medicine, Division of Renal Diseases and Hypertension. Dr. Monaghan received her Ph.D. in Cardiovascular Science at the University of Edinburgh, Scotland. Her doctoral research focused on the role of the blood and lymphatic microvasculature in the progression and regression of chronic kidney disease. Since her recruitment to the University of Colorado, her post-doctoral research position has focused on understanding the pathomechanisms that govern ADPKD and the potential role that adipocytes and adipose tissue immune cells may play in disease progression. Fundamentally, Dr. Monaghan’s work focuses on the development of overweight/obese ADPKD murine models in order to study mechanisms by which increased weight/adiposity drives the worsening of ADPKD. Further, development of such models will allow for the testing of new interventions aimed at lessening the burden of ADPKD and slowing cyst progression through either weight loss or targeted mechanistic mediations.
2023 research grant awardees
We are excited to share with you the ten grants and three fellowships selected for funding in 2023.
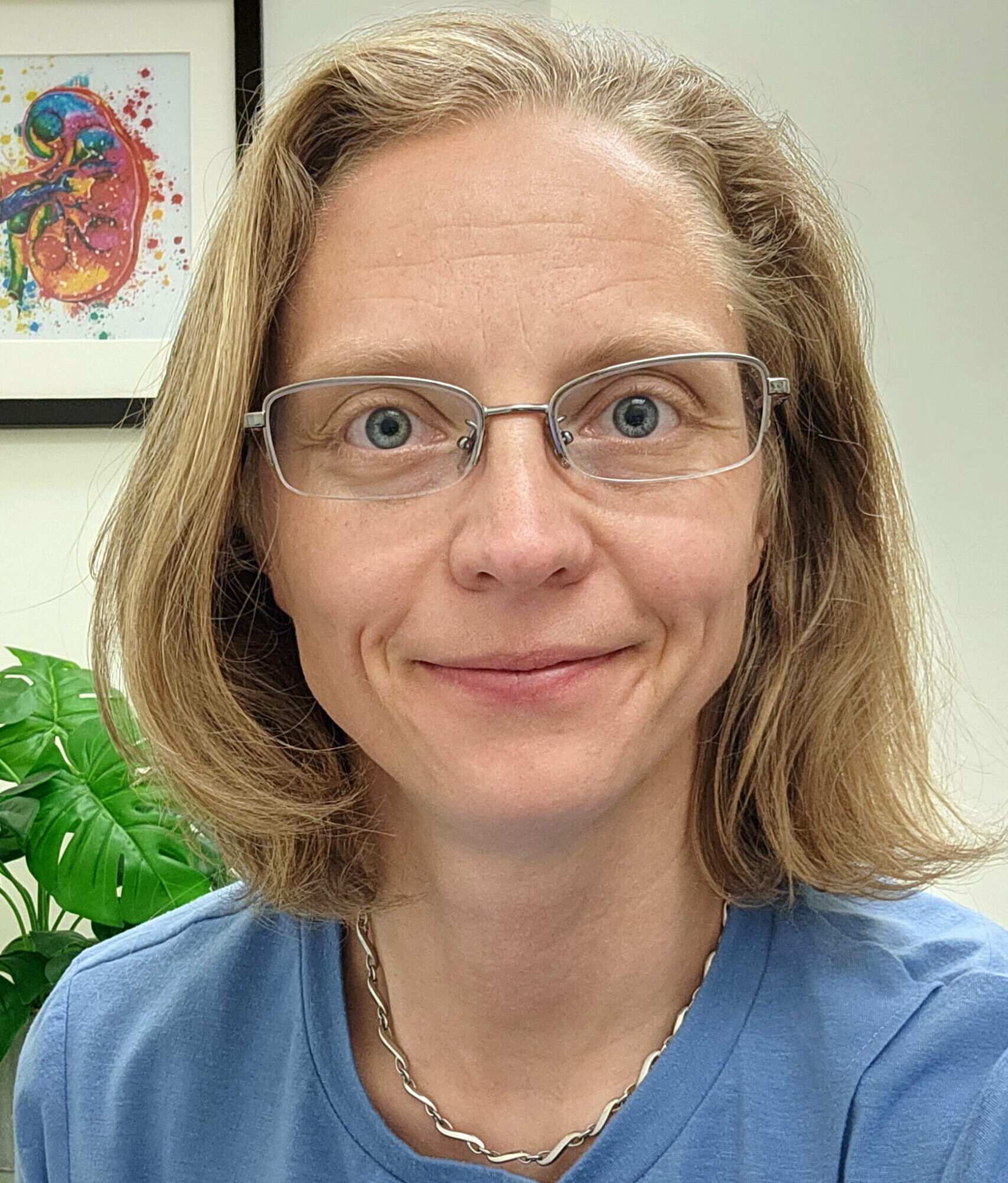
Katharina Hopp, Ph.D. - 2023 Dr. Vincent H. Gattone Research Award
University of Colorado Denver
Project Summary
GLP-1R agonists as novel therapeutic option for ADPKD
Studies of patients with overweight or obesity and Autosomal Dominant Polycystic Kidney Disease (ADPKD) show that body mass index (BMI) or abdominal fat mass are an important predictor of the rate of kidney cysts growth. Also, we recently found that efficacy of tolvaptan decreases with increasing abdominal fat mass. Hence, achievement and/or maintenance of a healthy BMI appears to be an important clinical target in the management of ADPKD. However, behavioral interventions to reduce BMI often fail due to low efficacy and challenges in adherence or tolerability. Further, weight loss is not a viable therapeutic option for patients with normal BMI and ADPKD as it provokes health risks such as bone loss, decreased immune function, or anemia, to name a few. Long-acting glucagon-like peptide 1 receptor agonists (GLP-1Ra[s]) are a highly effective next generation therapy that is changing management of patients with chronic obesity and/or type 2 diabetes. Beyond their weight loss and their regulatory role in glucose metabolism, GLP-1Ras have also been described to lower systemic and organ inflammation as well as cellular stress caused by reactive oxygen species. Since kidney cyst growth has been shown to be modulated by dysregulated glucose metabolism, inflammation, kidney immune cell function, and oxidative stress, we hypothesize that GLP-1Ras are an attractive and plausible therapeutic approach in ADPKD. Critically, this is independent of their effect on reducing BMI; meaning, we believe that GLP-1Ras may present a novel therapeutic approach even in patients with a healthy BMI, low abdominal adiposity, and ADPKD. In our preliminary studies we have established a diet-induced-obesity (DIO) ADPKD model using mice with a mutation to Pkd1 resulting in ADPKD phenotypes that mimic human disease. This DIO ADPKD model has significantly increased body weight and fat mass as well as more severe cystic kidney disease, paralleling the clinical findings. In addition, we found that DIO drives an increase in numbers of kidney immune cells that have been implicated to augment kidney cyst growth. In this application, we will test the therapeutic potential of semaglutide, a well-tolerated, FDA-approved GLP-1Ra, in slowing PKD progression using our established DIO ADPKD1 model. In addition, we will test semaglutide efficacy in the same ADPKD1 model, however, with mice being of healthy body weight. We will also include a group of mice which will receive no drug, but whose food intake is matched to that of the semaglutide group to control for the secondary effect that reduced food intake, known to occur with GLP-1Ra use, has on PKD progression. At the end of study, we will assess various commonly used preclinical parameters that establish severity of PKD which include total kidney volume measured by magnetic resonance imaging, kidney fibrosis and cystic burden evaluated on tissues sections of dissected kidneys, and kidney function. As a secondary outcome, we will also evaluate changes to vascular function using ultrasound imaging approaches that measure aortic stiffness. This secondary outcome is being considered because oxidative stress is known to impact cardiovascular health, which is compromised in patients with ADPKD and presents the most common cause of mortality. In addition, we will use a multitude of sophisticated methods that will allow us to understand the mechanisms through which semaglutide may impact PKD severity. These include assessment of abdominal adipose tissue function and secretion of proinflammatory factors, systemic and kidney metabolism, as well as kidney immune cell function and kidney- as well as vascular cell oxidative stress. Together, our study will be the first, to our knowledge, that tests the efficacy of a GLP-1Ra to slow kidney cyst growth using a clinically relevant disease model with or without obesity, building the foundation for clinical translation. The mechanistic studies will provide additional insight into relevant pathways that are modulated by GLP-1R signaling and may identify new alternate targets for treatment approaches as well as biomarker studies. Finally, our study will provide novel insight into the role of adipose tissue remodeling in PKD progression, an organ that has been poorly studied to date in PKD. All in all, this study addresses the PKD Foundation special consideration areas of “lifestyle interventions”, “PKD drug discovery”, and “extra-renal manifestations of PKD”.
Biography
Dr. Katharina Hopp, Ph.D., is an Assistant Professor at the University of Colorado Anschutz Medical Campus, Department of Medicine, Division of Renal Diseases and Hypertension. She has researched PKD pathomechanisms for the last 15 years predominantly utilizing rodent models. She completed her graduate work in Biochemistry and Molecular Biology with a focus on PKD genetics under the guidance of Dr. Peter Harris, Mayo Clinic, Rochester, MN. Since her move to CU Anschutz, her research program has focused on understanding the interplay between immune cells and the cystic epithelium. In particular, she is interested in understanding whether dysregulated metabolism, as characteristic for PKD, impacts immune cell function and if dietary/metabolism-based approaches can prime immune cells in functioning to slow kidney cyst growth. In her free time, Dr. Hopp enjoys exploring the Rocky Mountains with her family via camping, hiking, and running.
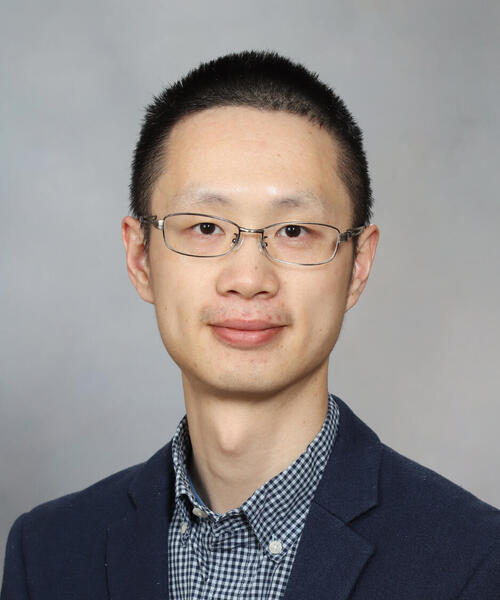
2023 Young Investigator Award - Kai He, Ph.D.
Mayo Clinic
Project Summary
Exploring the roles of axoneme polyglutamylation in the context of PKD
Inherited renal cystic diseases represent the most common human genetic diseases, including the ADPKD, ARPKD, the overlapping autosomal dominant polycystic liver diseases (ADPLD), and many syndromic forms of PKD, such as ciliopathies. ADPKD is the major form of PKD that mainly caused by mutations in PKD1/2 (encodes polycystin-1/2 or PC1/2) genes. Currently, tolvaptan, the only FDA-approved drug for ADPKD treatment, slows the disease by an indirect mechanism. However, it is only applicable for the patients with certain criteria and has substantial side effects. Optimal therapies capable of preventing diseases development or stopping their progression do not yet exist. Thus, drugs for treating PKD with wide spectrum of applicability and limited side effects are urgently needed.
Although the molecular mechanism of renal cystogenesis is still controversial, accumulating evidence suggest that the severity of PKD is closely correlates with the decreased functional dosage of polycystins. The primary cilium is a sensory antenna with central roles in sensing environmental cues and transduction of a variety of pivotal cellular signals. Polycystin complexes are hypothetically localize on primary cilia to inhibit renal cyst growth. Recent studies suggest that defective ciliary localization is one of the key mechanisms for decreased functional dosage of polycystins and cystogenesis in ADPKD, ARPKD, and PLD. Therefore, restoring the ciliary level of polycystins holds a strong therapeutic potential for these diseases but, unfortunately, without available molecular targets or drugs for that purpose.
Polyglutamylation (PG) is one of the tubulin posttranslational modifications that occur predominantly on cilia axoneme. Here, we propose that targeting axoneme PG could be a feasible and safe and therapeutic target for PKD for these reasons: 1) Axoneme PG controls the ciliary dosage of polycystins. 2) Increasing axoneme PG suppresses renal cyst growth in ex-vivo model of ADPKD. 3) Mouse genetic models with increased tubulin PG exhibit no noticeable physiological defects. 4) The proteins that control axoneme PG (enzymes, kinases, phosphatases) are conceptually druggable. However, there is no drug available to target axoneme PG and little is known about how axoneme PG is regulated.
Our preliminary studies identified a novel regulatory pathway for proper axoneme PG, targeting which increases ciliary polycystins and suppresses ex-vivo renal cystogenesis. Here, our proposed study aims to 1) further investigate the regulatory mechanism of axoneme PG. 2) screen small molecules, especially the existing clinical and preclinical drugs, to promote axoneme PG and increase ciliary polycystins. 3) determine the therapeutic effects of targeting axoneme PG in ADPKD. These studies will not only advance the knowledge of the regulatory mechanism of cilia function from basic science aspect, but also discover new targets and drugs to manipulate axoneme PG and ciliary polycystins. Importantly, completing the proposed studies could also discover a novel therapeutic strategy for PKD.
Biography
Kai He, Ph.D., is an Assistant Professor in the Department of Biochemistry and Molecular Biology at the Mayo Clinic. He received his Ph.D. in Biochemistry and Molecular Biology from the Shanghai Institute of Biochemistry and Cell Biology, Chinese Academy of Sciences, and postdoctoral training at the Mayo Clinic. He has received fellowship from the PKD Foundation, and the Outstanding Trainee Award from the Chinese American Society of Nephrology. Cilia-related diseases (ciliopathies) are life-threatening disorders that affect multiple organs. Manifestations include polycystic kidney and liver, obesity and diabetes, skeletal malformation, cardiovascular diseases, and brain anomalies. Despite the importance of primary cilia in human health, how cilia and ciliopathy protein function remains poorly defined. Dr. He’s research program has focused on the molecular basis of tightly regulated cilia function and its implications in ciliopathies. Dr. He also dedicates to identify small molecules to specifically target primary cilia and assess their therapeutic potential in the context of ciliopathies, especially in PKD.
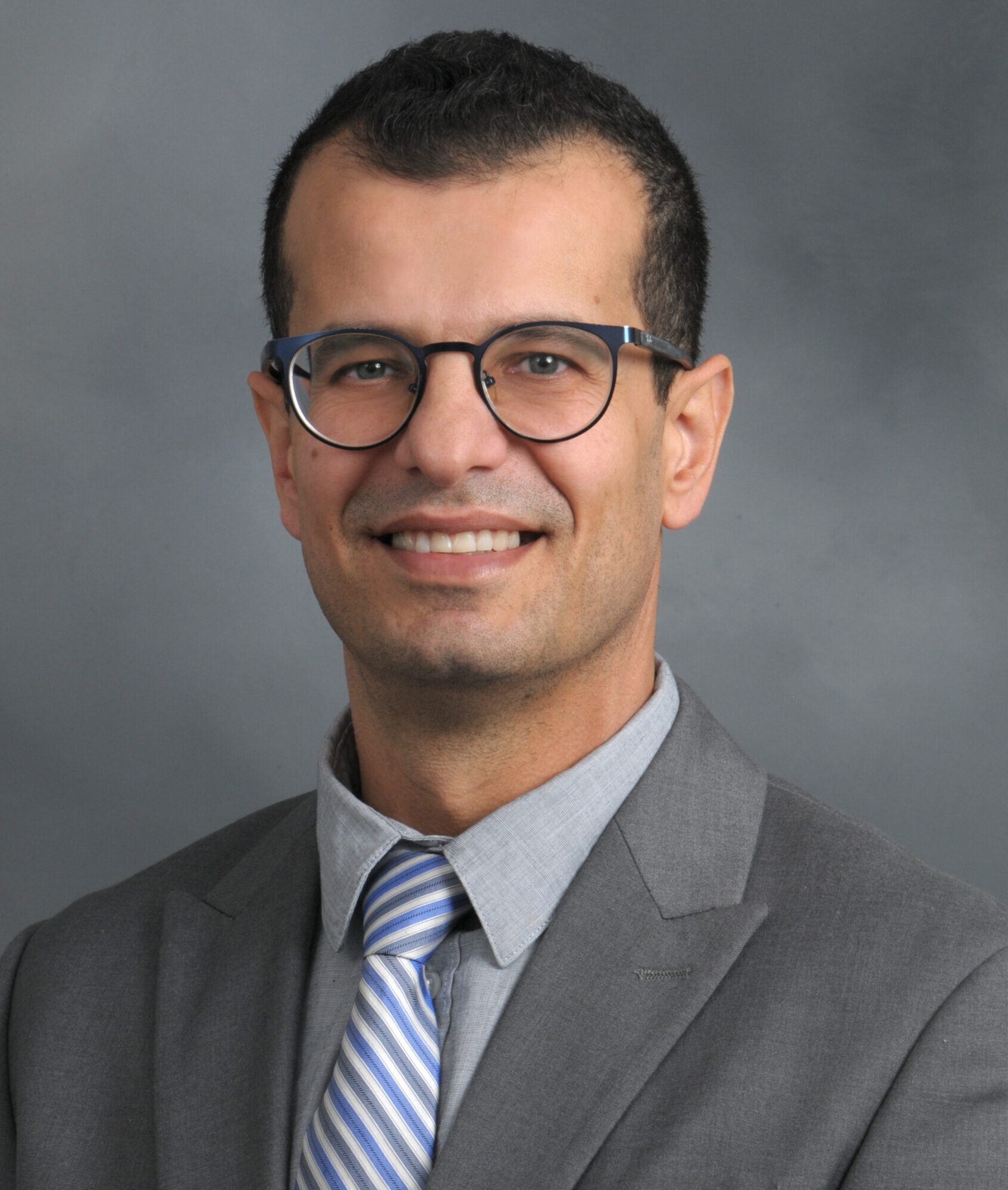
Karam Aboudehen, Ph.D.
SUNY at Stony Brook
Project Summary
Deciphering the role of the long noncoding RNA Pvt1 in ADPKD
Despite remarkable advances in our understanding of ADPKD in the last few decades, the disease still has a devastating impact on affected families and society in general. Every year, thousands of people are diagnosed with ADPKD worldwide; therefore, there is an urgent need to further investigate the molecular mechanisms and develop more effective therapies that can positively impact the life and health of individuals with ADPKD. Long noncoding RNAs (LncRNAs) are a class of non-protein coding RNAs with pivotal functions in development and disease. They have emerged as an exciting new drug target category for many common conditions. However, the role of lncRNAs in ADPKD has been understudied. This proposal will determine the impact of a known, highly conserved, and pathogenic lncRNA, called PVT1, on ADPKD pathogenesis. Our proposed assays are based on strong preliminary data showing that Pvt1 silencing reduces cyst growth in cultured kidneys from Pkd1-null mouse embryos. We will utilize pre-clinical models of mouse and human ADPKD to determine whether inhibition of PVT1 ameliorates cystogenesis. One protein that is known to be regulated by PVT1 and plays a critical role in advancing ADPKD is c-MYC. c-MYC is an attractive drug candidate; however, targeting c-MYC remains a challenge. Our proposal provides a complementary approach to traditional drug development in ADPKD. This approach focuses on targeting a single lncRNA to manipulate multiple genes, proteins, and pathways. Should we succeed, it would open the door for exploring the therapeutic potential of inhibiting PVT1 and reducing c-MYC levels to ameliorate cystogenesis in ADPKD.
Biography
Karam Aboudehen is an Assistant Professor in the Department of Medicine, Division of Nephrology, at Stony Brook University (SBU). In 2012, he completed his graduate training at Tulane University under the guidance of Dr. Samir El-Dahr in Pediatric Nephrology. He then joined Dr. Peter Igarashi laboratory as a post-doctoral fellow at the University of Texas Southwestern Medical Center in Dallas. In 2016, Dr. Aboudehen was appointed to a faculty position at the University of Minnesota before recently joining the Nephrology Division at SBU in 2022. Research in Aboudehen’s lab focus on investigating the role of long noncoding RNAs (lncRNAs) in polycystic kidney disease (PKD). The long-term objective is to discover therapeutically targetable lncRNAs that prevent or mitigate cyst formation and/or progression in PKD. His lab utilizes cutting edge technologies that include mouse molecular genetics, next generation sequencing and mass spectrometry, genetic engineering, RNA pulldown assays, and viral gene delivery.
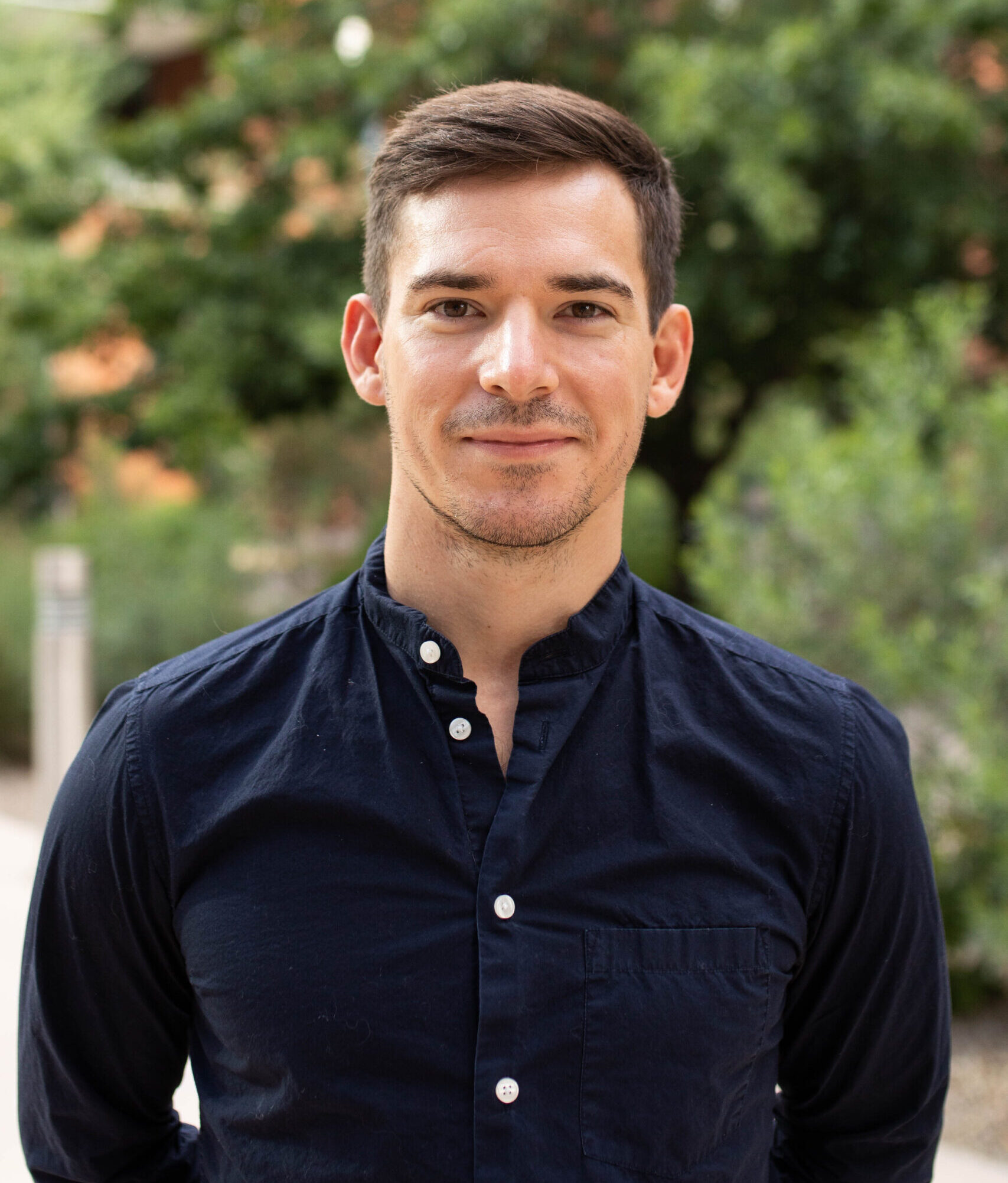
Christopher Banek, Ph.D.
University of Arizona
Project Summary
Renal Innervation and Nerve Activity Influence on Cystic Progression in ARPKD
Polycystic kidney disease (PKD), both autosomal dominant (AD) and autosomal recessive (AR) forms, remains the leading cause of inheritable kidney disease in adults and children throughout the world. PKD is defined by the development of non-cancerous neoplasms (i.e. fluid-filled sacs) that grow overtime and progressively cause kidney failure. Though the cause of renal cyst formation is well-understood to be primarily driven by an inherited or spontaneous genetic mutation, there remains no cure beyond kidney transplantation. Since access to kidney transplantation is extremely limited, it is vital to this patient population to study alternative strategies for treatment. In recent years, there has been a very important advancement in drug therapy, known as Tolvaptan, that interrupts the vasopressin type 2 receptor (V2R) in the kidney. This receptor contributes to cyst growth in PKD, and Tolvaptan has been shown to slow cyst progression and, in turn, kidney failure. While promising Tolvaptan serves as an excellent intervention currently, there are notable side effects that decrease a patient’s quality of life, such as excessive thirst (polydipsia) and urination (polyuria) – all day and all night. Our laboratory is focused on targeting the same pathway through the kidney’s nervous system, which is known to regulate kidney function and potentially V2R signaling.
The kidney is innervated with many nerves that relay signals to the kidney from the brain (efferent nerves), and from the kidney to the brain (afferent nerves). In several preclinical studies in other models of kidney disease, the activity of these kidney nerves is increased compared to healthy controls. If these signals are disrupted by surgically cutting these nerves, many models of kidney disease are improved. This procedure is referred to as renal (i.e. kidney) denervation (RDNx). Most excitingly, our lab has recently reported that performing the RDNx procedure in a rat model of PKD slows cyst growth. Notably, there were no effects on water intake or urination volumes, which may be preferential to the side effects noted earlier with Tolvaptan. While these early results are promising indeed, further research is required to understand the molecular pathways affected by the RDNx procedure before advancing this new treatment to clinical trials.
A few outstanding questions remain regarding the effect of RDNx. Firstly, it remains unknown if there are direct effects on the V2R signaling in the kidney. The experiments outlined in our proposal directly test this to see if this receptor is involved in the effects of RDNx. Secondly, it is unknown if kidney nerve activity is increased progressively as the cysts grow. We have carefully designed experiments to quantify the nerve signaling to determine when and if nerve activity is altered. This would be very valuable in determining when the RDNx procedure would be most effective. Finally, we will determine the if the beneficial effects of RDNx are dependent on the removal of the local proteins that are released at the ends of these nerves (i.e. neurotransmitters). This will determine whether the signal relayed to the brain is more important, or if the neurotransmitters contribute to the cyst progression.
Overall, Dr. Banek’s laboratory is well-experienced and equipped to run these studies at the University of Arizona and Sarver Heart Center. These studies are carefully designed to reveal the underlying mechanisms of the kidney’s nervous system and its contribution to PKD progression. If successful, RDNx and/or the underlying mechanistic targets may be quickly launched to the next stage for clinical translation to complement the severely limited tool chest for PKD treatment.
Biography
Christopher Banek, PhD is an Assistant Professor in the Department of Physiology at the University of Arizona College of Medicine in Tucson, AZ. Dr. Banek has an expansive training background in neuro, cardiovascular, and renal physiology, with a specific emphasis on hypertension and renal injury/inflammation. He received his PhD in Human Physiology from the University of Oregon, and completed a postdoctoral fellowship in cardiovascular physiology at the University of Minnesota Medical School. Throughout his career, Dr. Banek has focused on the physiological underpinnings of cardiovascular and renal disease – largely in the context of high blood pressure (i.e. hypertension) and kidney dysfunction. His recent studies have largely focused on the contributions of the kidney’s nervous system to the development and progression of polycystic kidney disease. Dr. Banek’s team recently published a study on the novel application of a targeted nerve ablation technique (renal denervation; RDNx) that disrupts the kidney’s nervous system in a model of autosomal recessive PKD, which reduced kidney cystic progression and blood pressure. Dr. Banek and his team are now focused on elucidating the underlying mechanisms and role of renal nerves in the progression of renal cysts, as well as the potential for RDNx as a novel therapeutic modality for PKD intervention.
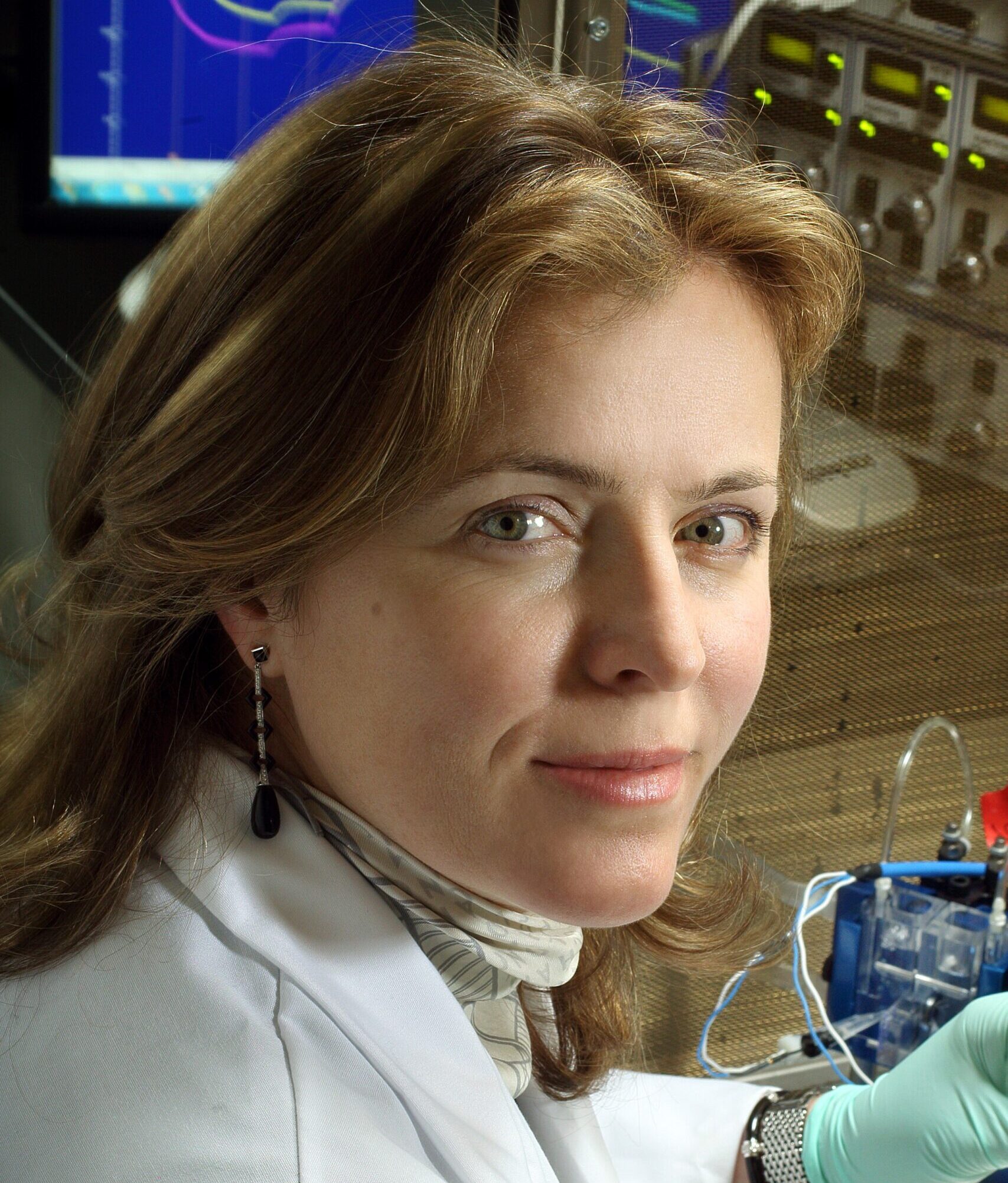
Liudmila Cebotaru, M.D., J.D.
Johns Hopkins University School of Medicine
Project Summary
Developing a New Therapeutic Approach for Autosomal Recessive Polycystic Kidney Disease
Autosomal recessive polycystic kidney disease (ARPKD), caused by mutations in the PKHD1 gene (2,3), is a debilitating genetic disorder causing newborn morbidity and mortality. The most severe disease occurs when the fetus develops abnormal kidneys and a deficit in fluid surrounding the fetus (4). Those who survive to birth face a myriad of symptoms, including increased blood pressure and changes in the fine structure of the liver; they also develop dilations in portions of the kidney ultimately leading to its destruction (5). Management of the disease in infants who survive the first weeks of life is difficult, requiring extreme measures including kidney and/or liver transplant (6). Clearly, there is a critical need to develop treatments for ARPKD. We provide compelling data showing that an approved drug for cystic fibrosis, VX-809, reduces abnormal cyst growth in liver cells from ARPKD model mice. We propose the novel hypothesis that drugs used to treat cystic fibrosis can be used as a treatment for ARPKD and (7) can be fast-tracked for ARPKD treatment. The goal here is to provide a strong proof-of-principle and mechanistic background that will allow the drugs to move forward as a treatment for ARPKD and to establish a new paradigm based on restoring key mechanisms to reduce disease in the lungs, kidneys, and liver. Aim 1 will address the therapeutic potential of cystic fibrosis drugs by determining how they restore function in multiple models of ARPKD. Here we will evaluate a new therapeutic paradigm, using two mouse models to assess the ability of drugs to restore liver and kidney function to these animals. These experiments in rat and mouse models will provide the proof-of-principle that can be used to develop modulators into approved drugs for the treatment of ARPKD. Aim 2 will uncover novel mechanisms that lead to multiorgan disease in ARPKD. We have found three profound bodily functions that misfunction in ARPKD, which together may be targets for therapeutic intervention. In this Aim, we will address the contributions of each of these to the pathology associated with ARPKD. The goal is to define pathways in the body that lead to disease, thereby providing a mechanistic understanding of how modulator drugs work to reduce the dis-ease. An understanding of drug mechanism is not only important for clinical development but also for the development of additional medicines that might work even better.
Biography
Dr. Liudmila Cebotaru’s training in medicine began at the age of 18, when she received a R.N. degree from Medical College of Balti, Moldova. She continued her medical training by obtaining an M.D. degree from University of “Carol Davila” School of Medicine and Pharmacy of Bucharest, Romania, and then obtained further training in the areas of pathology. While studying medicine, she also successfully achieved a J.D. degree from the University of Bucharest, Law School and continued in the US, where she later received a Master’s Degree in US Law from the University of Baltimore. Her training in the U.S. began as a post-doctoral fellow at Johns Hopkins University in Gastroenterology and Physiology. She remained at JHU and was promoted to Associate Professor in 2017. In keeping with her training in both medicine and basic science, her passion is to understand the molecular basis of the disease process and to restore normal function to disease-causing mutant proteins. She strongly believes that by understanding the mechanism of the disease process at a basic level, we can devise strategies to correct or at least, bypass defective proteins and restore function. She is currently conducting extensive studies in developing gene therapies for Cystic Fibrosis and in the role of CFTR in Autosomal Dominant Polycystic Kidney Disease. As a result of her past studies on the mechanism of how the cell handles defective proteins and how to rescue function using CFTR modulators, she uncovered a potential new strategy for reducing liver and kidney disease in Autosomal Recessive Polycystic Kidney Disease.
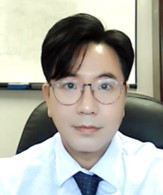
Harrison Kim, Ph.D.
University of Alabama at Birmingham
Project Summary
Accurate assessment of intrarenal perfusion in ADPKD using quantitative DCE-MRI
To date, the best methods of identifying high-risk patients for rapidly progressive ADPKD are based on total kidney volume (TKV). However, TKV trajectories are typically not smooth even when followed yearly, and an accurate assessment of therapeutic response based on TKV may require several-year follow-ups. Thus, there is an urgent need to develop a more sensitive alternative for disease activity monitoring.
Intrarenal perfusion may serve as a robust indicator of ADPKD severity. ADPKD causes polycystin impairment in the renal endothelial cells, leading to vascular remodeling, ischemia, fibrosis, and, consequently, perfusion decrease. Dynamic contrast-enhanced magnetic resonance imaging (DCE-MRI) can non-invasively assess tissue perfusion by monitoring the dynamic change of MRI contrast agents. Macrocyclic gadolinium-based MRI contrast agents (e.g., gadoteridol) are known to be safe even in patients with stages 4 and 5 of chronic kidney diseases. The volume transfer constant (Ktrans), one of the quantitative DCE-MRI (qDCE) parameters, measures tissue perfusion.
However, the measurement variability across different MRI scanners remains a concern for the reproducible application of qDCE across various clinics. Each MRI vendor provides unique hardware configurations, pulse sequences, and reconstruction algorithms, which cause variations in quantitating tissue contrast agent concentration and DCE-MRI parameters. An external phantom with a known contrast agent concentration may allow us to detect and correct the variation. Furthermore, if the phantom is miniaturized to be imaged concurrently in the bore of a standard MRI scanner with a patient, the intra-scanner variability due to hardware instability can also be reduced.
We developed a point-of-care perfusion phantom named P4 (Point-of-care Portable Perfusion Phantom). The P4 phantom can be imaged with a human subject in a standard MRI scanner to reduce inter/intra-scanner variabilities. In our recent study, the intraclass correlation coefficient (ICC) of Ktrans measurement across three 3T MRI scanners in two clinics was improved from 0.38 to 0.99 after the P4-based error correction. The P4 phantom is inexpensive and ready to use in any commercially available MRI system without needing adjustment. Therefore, the P4 phantom is ideal for highly reproducible qDCE measurement of intrarenal perfusion.
We hypothesize that the intrarenal perfusion measurement using qDCE is highly reproducible when a P4-based error correction scheme is employed. We aim to test this hypothesis with an ADPKD patient cohort at the University of Alabama at Birmingham (UAB). The anticipated high reproducibility of the P4-based qDCE measurement will provide strong evidence for integrating this method into ADPKD clinical trials as a novel phenotypic readout. If successful, this technology will: a) improve precision in ADPKD risk stratification and prediction of outcomes, allowing more timely dose adjustment of therapeutics, and b) enable accurate data comparison across different MRI scanners, facilitating collaboration among institutes to develop advanced treatments for this devastating disease.
Biography
I am a professor in the Department of Radiology for the Division of Advanced Medical Imaging Research at the University of Alabama at Birmingham (UAB). My research vision is to globally standardize quantitative imaging of various diseases including polycystic kidney disease (PKD), which will facilitate automatic prognosis and therapy monitoring for patients. Automatic clinical decisions will drastically reduce the turnaround time and medical expenses for patients. My research mission is to develop hardware and software tools along with this initiative and validate those tools in multi-institutional clinical trials. In the proposed study, I will develop a novel prognostic imaging biomarker for PKD, which will be used for early therapy adjustment.
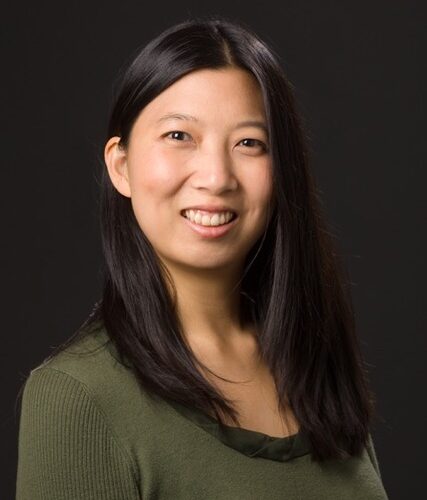
Ivana Kuo, Ph.D.
Loyola University Chicago
Project Summary
Cardiac-driven hypertension in Autosomal Dominant Polycystic Kidney Disease
Heart failure is the main cause of death in patients with Autosomal Dominant Polycystic Kidney Disease (ADPKD). One of the most significant preventative measures to reduce heart failure is to lower blood pressure. This is because the heart is a pump, and when the hose (blood vessels) become too tight, the buildup in pressure causes the pump to fail (i.e.: heart failure). High blood pressure (hypertension) affects over 70% of all ADPKD patients and is one of the earliest detectable symptoms of ADPKD.
Almost all ADPKD patients take medications that reduce blood pressure. However, many factors cause hypertension. These include things we can put into our body, like a high salt diet, or things inside our bodies, like a cystic kidney pushing on the kidney blood vessels. The kidney cysts start a vicious cycle where the kidneys release of a substance called renin. Renin causes the production of substances that cause the body to hold onto more salt, resulting in an even higher blood pressure. Most ADPKD patients receive medications called ACE inhibitors or ARBs that try to break the renin cycle and lower blood pressure. However, the heart can also release helpful factors, called natriuretic peptides, that lower a patient’s blood pressure. Natriuretic peptides are the body’s own way of trying to fix hypertension as they allow the heart and blood vessels to relax (thus reducing blood pressure) and cause the kidneys to excrete more salt in the urine. These combined effects of relaxation and decreased salt all reduce blood pressure. Our end goal is to see if we can help the hearts of ADPKD patients help their kidneys by producing more beneficial natriuretic peptides and ultimately reduce hypertension and heart failure.
Our mice which do not have polycystin 2 (one of the two genes which when mutated result in ADPKD) in the heart but have normal kidneys have profound hypertension. Excitingly, our studies suggest that the hypertension happens because the mice without polycystin 2 in the heart cannot produce natriuretic peptides. In this proposal, we have two goals that will help us better understand how loss of polycystins in the heart leads to hypertension and if existing FDA approved treatments that preserve natriuretic peptides can reduce hypertension in ADPKD.
Our first goal is to better understand how loss of polycystin proteins prevent the production of natriuretic peptides. We will test if polycystin 1 and polycystin 2 (the two genes which when mutated cause the majority of ADPKD) both contribute to the production of natriuretic peptides. Using modified heart cells grown in the laboratory, we will test different mutations to polycystin 1 and 2 that occur in ADPKD patients for their ability to produce natriuretic peptides. The impact of completing this goal is that we will know if patients with polycystin 1 or polycystin 2 mutations both have reduced natriuretic peptide production. This may help inform how a patient’s hypertension is developing and provide clinicians with opportunities to intervene.
Our second goal is to test in our hypertensive mouse if an already approved heart failure FDA drug (sacubitril/valsartan; marketed by Novatis as Entresto) reduces blood pressure. Entresto is a dual formation drug that keeps natriuretic peptides from breaking down in the body (sacubitril) and an ARB that targets the renin pathway (valsartan). This dual combination treatment may be more effective than the renin pathway drugs that are commonly prescribed to ADPKD patients.
For the ADPKD patient, these studies will be the first scientific test of how the polycystin proteins decrease natriuretic peptide production, and second, these studies will test an already approved FDA natriuretic peptide drug to reduce blood pressure. Ultimately, the hope is that we will have better hypertension treatments that also reduces heart failure in the ADPKD patient.
Biography
Dr. Ivana Kuo is an Assistant Professor in the Department of Cell and Molecular Physiology at Loyola University Chicago. She obtained her PhD in Neuroscience at the Australian National University, before studying the function of polycystin proteins in her postdoctoral work at Yale University in the Department of Pharmacology under the mentorship of Dr. Barbara Ehrlich. Dr. Kuo’s laboratory uses cell and mouse models to understand the cardiovascular manifestations within ADPKD, with a particular emphasis on how polycystin proteins in cardiomyocytes contribute to cardiac function.
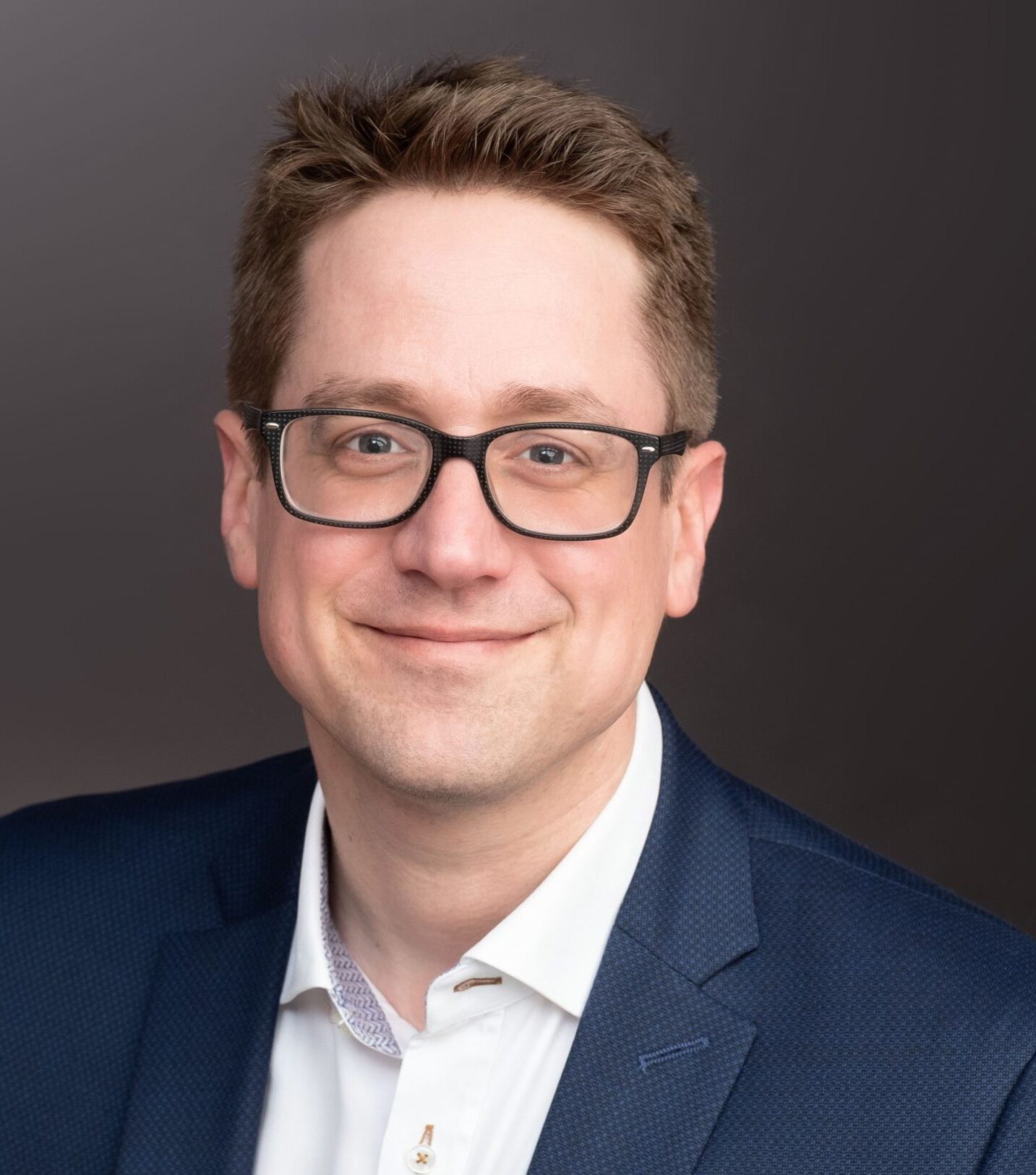
Max Christoph Liebau, M.D.
University Hospital Cologne
Project Summary
Identifying clinical and biochemical risk markers of ARPKD liver disease
Autosomal recessive polycystic kidney disease (ARPKD) is a disorder, that typically presents with symptoms in childhood. The two organs that are mainly affected are the kidneys and the liver. Kidney disease in ARPKD results in enlarged cystic kidneys and impaired kidney function. Liver disease in ARPKD is characterized by inborn fibrotic changes of the liver and dilated bile ducts. While the fibrotic changes lead to congestion of the blood flow through the liver, the dilated bile ducts result in an increased risk of severe infection and inflammation for patients. Liver disease in ARPKD may result in a need for patient to undergo liver transplantation or combined liver and kidney transplantation. ARPKD is one of the two main reasons for combined liver and kidney transplantation in childhood.
Different from kidney disease in ARPKD, first symptoms associated with liver disease in ARPKD tend to present a bit later in childhood and may show progression until adulthood. For some patients the involvement of the liver may then even be more severe than kidney disease. However, it is not well-known why some patients with ARPKD develop a more pronounced form of liver disease than others and if there are early clinical, imaging or laboratory findings or disease patterns that could indicate a high risk to patients and caregivers at an early timepoint in life. It is also not well-understood, how different variants in the ARPKD-causing gene PKHD1 lead to liver disease.
In the past we have mainly studied risk factors of kidney disease progression in ARPKD. We identified first risk markers of rapid progression of kidney disease n childhood. These studies have facilitated the establishment of first clinical trials.
In the current project we aim to follow the same path for liver disease. We will use the existing data of more than 700 patients in the European ARegPKD registry, the largest clinical dataset on clinical longterm courses of patients with ARPKD collected so far. Using complex statistical workup methods we aim to identify risk markers at an early timepoint in young childhood that could help to predict the progression of liver disease. This could include ultrasound or other imaging findings, laboratory values, specific genetic variants or a combination of different markers.
In addition to the statistical workup of the clinical findings, we aim to identify blood markers of liver disease in ARPKD. In previous ARegPKD analyses we identified PKHD1 variants that are associated with variable degree of liver disease. We have used the novel molecular technique of CRISPR/Cas9 and have generated corresponding mouse models carrying exactly the same variants. We will characterize disease progression in these mouse models by studying liver tissue structure and protein expression profiles at different time points in an independent project. Bridging the work between clinical markers and laboratory studies we will now study potential novel serum biomarkers in the here suggested study. We have successfully established the technique of unbiased serum proteome analyses in Cologne and have deep pre-existing data on typical patterns in children with chronic kidney disease. For these studies a volume of 5µl is enough to perform the highly-specific analyses of around 300 proteins in serum at the same time. Serum proteins serve as biomarkers for many diseases and we hypothesize that there may be specific changes of serum markers due to liver disease in ARPKD that we can identify through this unbiased approach. We also hypothesize that early markers can differentiate between the disease patterns and that they can be helpful add-ons to clinical markers. Using the newly developed mouse models, we will analyse how serum proteome patterns change over time in mice with liver disease and/or kidney disease. In addition to studying the more clearly defined mouse models we will also perform proteome analyses of patient samples and will study associations with the clinical extent of liver and/or kidney disease documented in ARegPKD. In complex bioinformatical approaches we will integrate the findings from clinical observations and serum proteome measurements in a joint model that will help to improve prediction profiles for individual patients at a young age childhood.
This project could have impact for ARPKD research in multiple ways. It could help to identify subgroups of patients with liver disease that could be studied and compared more precisely in clinical trials. Here, clinical and biochemical markers could be combined and the risk for severe liver disease may be predicted more precisely thus supporting clinical decision-making. The project could also help to establish novel models to specifically study molecular mechanisms of liver disease development and progression in ARPKD, which could be helpful for future studies of therapeutic approaches.
Biography
Max C. Liebau, MD, is a clinical consultant pediatric nephrologist at the Department of Pediatrics at the University Hospital Cologne, Germany, where he holds positions as Head of the Social Pediatric Center for Chronically Ill Children and Head of Translational Pediatric Nephrology. Dr Liebau combines his clinical training as a pediatric nephrologist with his experience in cellular and molecular biology obtained in the Nephrology Research Laboratories in Freiburg and Cologne, Germany and at the University of California, Santa Barbara. His group follows a translational research approach to study genetic kidney diseases with a special focus on Autosomal Recessive Polycystic Kidney Disease (ARPKD). The group aims to understand the molecular function of the ARPKD protein fibrocystin and to characterize clinical long-term courses of ARPKD as a basis for the identification of clinical and/or biochemical risk markers of disease progression. Dr Liebau initiated and is currently leading the international ARPKD registry study ARegPKD and is a co-initiator of the pediatric ADPKD registry study ADPedKD. His research is funded by the German Research Council and the German Federal Ministry for Education and Research amongst others.
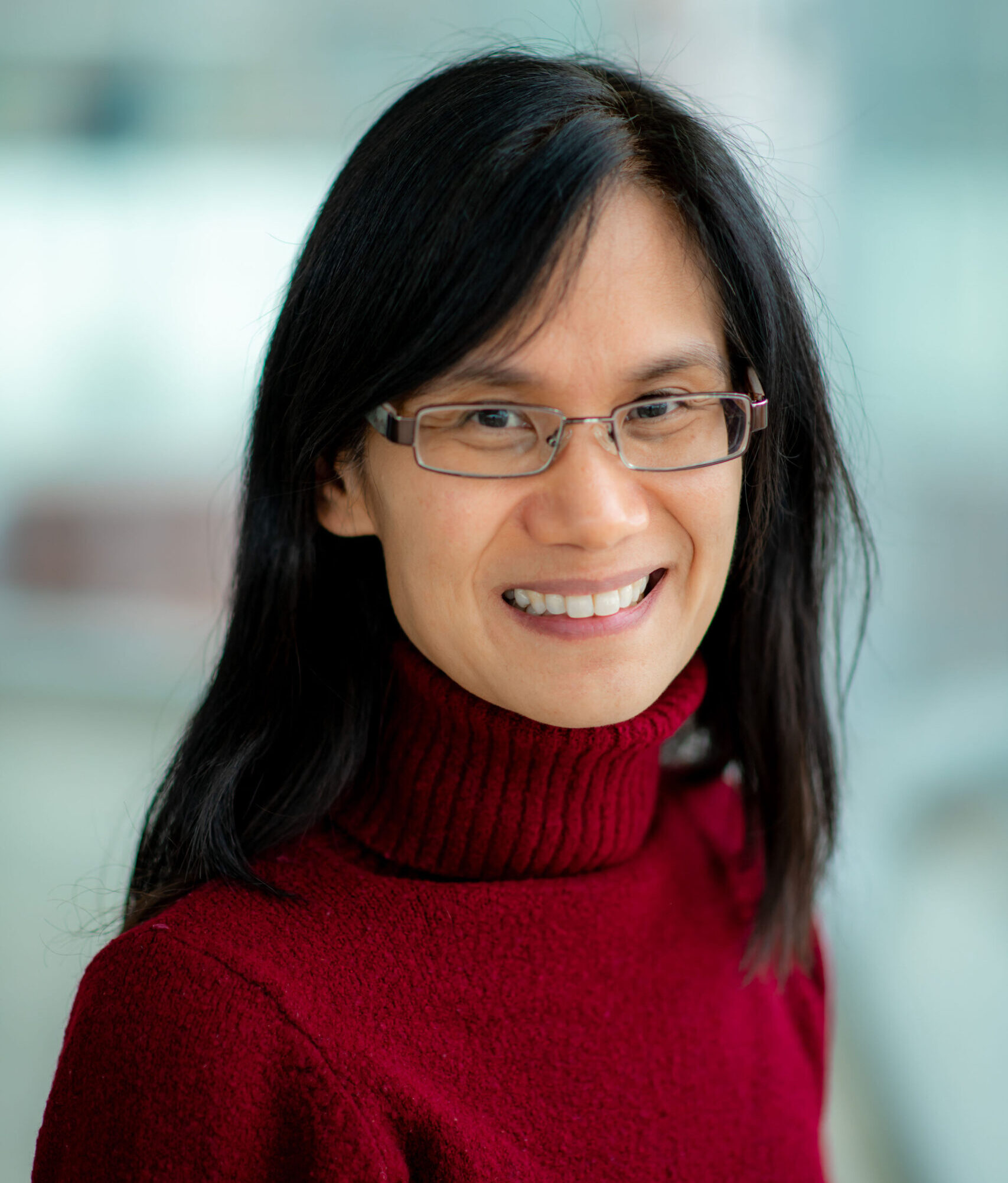
Pamela Tran, Ph.D.
University of Kansas Medical Center
Project Summary
A novel nutrient sensor pathway in ADPKD
Autosomal Dominant Polycystic Kidney Disease (ADPKD) is among the most common, life-threatening hereditary kidney diseases affecting approximately 1:1000 individuals worldwide. ADPKD causes progressive growth of large fluid-filled renal cysts, which causes kidney injury and can lead to end-stage renal disease. The disease also causes pain, hypertension, bladder infection, vascular complications and liver cysts. As such, the medical burden of ADPKD affects quality of life. There is one FDA-approved therapy, but it has variable effectiveness and multiple side effects. Thus, there is a need to increase our understanding of the mechanisms that drive ADPKD progression to devise new therapeutic strategies. The molecular etiology of renal cystogenesis is complex, but studies indicate the disease mechanism originates from a defect in the primary cilium. The primary cilium is a sensory organelle that converts chemical and mechanical cues into pathways that regulate cell homeostasis. Altered cellular metabolism has emerged as an important contributor to ADPKD pathogenesis, and studies suggest primary cilia and cellular metabolism connect. We propose that a nutrient sensor pathway acts both as a novel potential therapeutic target and molecular link between the ciliary and metabolic defects in ADPKD. Using a mouse model that mimics the slowly-progressive nature of the disease as well as our recently developed cell culture device that enables formation of in vitro 3D renal tubules comprised of patient-derived cells to evaluate the chemosensory function of ADPKD primary cilia, we anticipate our experiments will show that ADPKD renal cystogenesis is mitigated by inhibition of this nutrient sensor, and that novel regulatory mechanisms, feedback loops, and in turn, additional therapeutic targets will be revealed. Our 3D renal tubule mimetic creates a more physiologically relevant environment and can be used for future screening of pharmacological compounds. Thus, completion of this proposal will present a new therapeutic target and novel avenues to identify additional therapeutic strategies against ADPKD. This will contribute toward alleviating the immense medical burdens of this common genetic disease.
Biography
Pamela Tran, PhD, is an Associate Professor in the Department of Cell Biology and Physiology and in the Jared Grantham Kidney Institute at the University of Kansas Medical Center (KUMC). She is Co-Director of the In Vivo Models Core of the PKD-Research Resource Consortium at KUMC. For 20 years, she has been using genetic mouse models to investigate the role of primary cilia dysfunction in various diseases, such as PKD, obesity and skeletal disorders. In collaboration with other investigators at KUMC, her laboratory has found that a post-translational modification and regulator of metabolism, O-GlcNAcylation, is upregulated in ADPKD. This proposal seeks to explore a possible connection between increased O-GlcNAcylation and the ciliary defects in ADPKD.
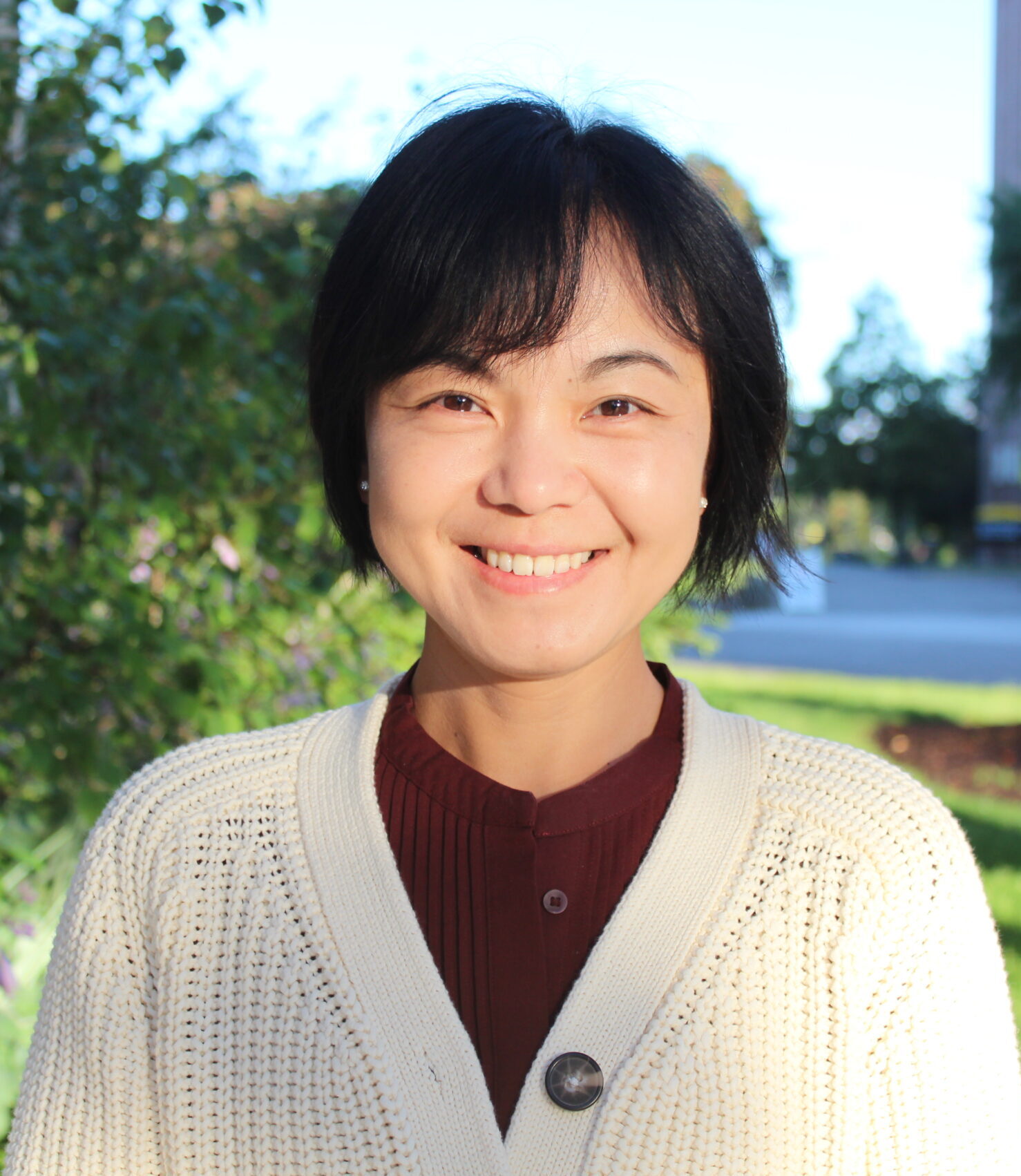
Yan Zhang, Ph.D.
Michigan Technological University
Project Summary
The role of high mobility group box 1-induced NF-kappa B activation in polycystic kidney disease
Autosomal dominant polycystic kidney disease (ADPKD) is characterized by the formation and growth of numerous fluid-filled cysts and the development of tissue inflammation and fibrosis. Inflammation is a key driving force for cyst growth and tissue fibrosis of ADPKD. This study will help to understand the molecular mechanisms for the inflammation development of ADPKD and will test resolvins, newly discovered anti-inflammatory molecules, as a potential therapeutic approach to slow the disease.
Biography
Yan Zhang is a Research Assistant Professor in the Department of Biological Science at Michigan Technological University. Dr. Zhang earned her PhD in Pharmacology and Toxicology from the University of Missouri-Kansas City, and her work investigated the molecular mechanisms mediating “sterile” inflammation, demonstrating that toll-like receptors are required for endogenous damage-associated molecular patterns-mediated activation of innate immunity. After that, Dr. Zhang completed her postdoc training in Dr. Darren Wallace’s laboratory of Jared Grantham Kidney Institute at the University of Kansas Medical Center. Her research has focused extensively on identifying key regulators for cyst growth, interstitial inflammation, and fibrosis in PKD and novel therapeutic approaches to slow the disease. Her work showed that conditional overexpression of transforming growth factor-beta1 in collecting ducts increased cell proliferation, accelerated renal fibrosis and the decline of renal function, and shortened the lifespan of PKD mice. Her work also demonstrated that elevated Ca/Calmodulin-dependent kinase 4 in cystic epithelial cells is a novel upstream regulator of mTOR, a key regulator of cell proliferation and cyst growth in ADPKD. In addition, she examined the role of liver kinase B1 (LKB1), the primary upstream regulator of AMP kinases, in the disease progression of ADPKD and found that LKB1 is an important regulator of key pathways involved in cyst growth and fibrosis, and activation of LKB1 slows disease progression of PKD mice.
2023 fellowships
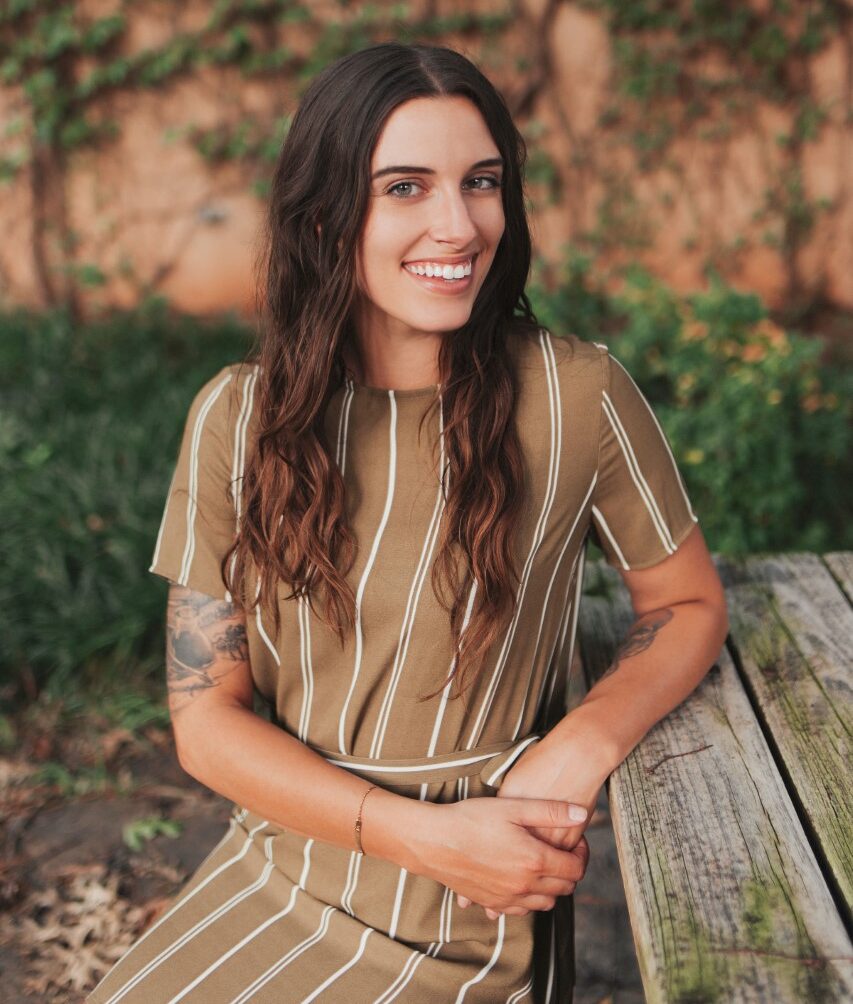
Sarah Miller, Ph.D.
University of Oklahoma, Health Sciences Center
Project Summary
The Role of Trem2+ Cyst Associated Macrophages in Polycystic Kidney Disease
Polycystic kidney disease (PKD) affects over half a million people in the United States and is one of the most common genetic kidney diseases. The disease is characterized by gradual development of kidney cysts throughout a patient’s lifetime, which eventually leads to end-stage kidney disease requiring renal replacement therapy or transplant. The slow progressing nature of autosomal dominant PKD (ADPKD) means there are opportunities for pharmacological or behavioral interventions aimed at slowing disease progression. Data from multiple studies indicate that a type of immune cell in the kidney, called macrophages, promote cyst progression in animal models of the disease. However, the majority of data that supports these findings was collected using rapidly progressing congenic (i.e. born with the disease) or injury accelerated (i.e. mice are given a renal injury to make cysts grow faster) models of disease. This equates to studying the role of macrophages during 0-10 (congenic) or 16-24 years of age (injury accelerated) in humans. This does not accurately reflect the timing (age of cyst onset) or rate of disease progression (slow) in humans, where cysts begin to enlarge in the 2nd decade of life and gradually progress until end stage renal disease is reached in the 5th-9th decade. In preliminary studies, I analyzed the gene expression profiles of kidneys from both slowly and rapidly progressing models of cystic disease as well as non-cystic controls. My data identified a major difference in the macrophage profile between slow and rapid cystic models and non-cystic controls. This included a population of cyst associated macrophages (CAM) that were only present in the slowly progressing model that expressed the gene Trem2. Based on data showing a protective role for Trem2+ macrophages in other slowly progressing diseases, I hypothesize that Trem2+ CAMs restrict cyst growth. In this project I will use a slowly progressing mouse model (Pkd1RC/RC) that develops cystic disease at an age and rate similar to patients to determine the role of Trem2+ CAMs in a clinically relevant animal model. I will also test whether Trem2+ CAM macrophages are present in kidneys isolated from end stage ADPKD patients. Thus, these studies will challenge the central paradigm that all kidney macrophages promote PKD progression, and instead pursue the concept that there are beneficial subpopulations of macrophages that can help restrict cyst growth. The idea of enhancing protective macrophages which may directly inhibit cyst growth has the potential to be of significant translational value to the field as antibodies that enhance Trem2+ CAM activity are currently in clinical trials for patients with Alzheimer’s disease and may be rapidly repurposed for patients with ADPKD.
Biography
I am postdoctoral researcher working under the mentorship of Dr. Kurt Zimmerman in the Department of Internal Medicine, Division of Nephrology at the University of Oklahoma Health Sciences Center (OUHSC). During my graduate training in the lab of Dr. Jimmy Ballard, my work was primarily focused on the large clostridial toxin TcdB, produced by the bacteria Clostridioides difficile. During my studies, I found a 19-amino acid region in the toxin that, when deleted, prevented membrane translocation while leaving the toxin enzymatically active. This toxin mutant was used as a vaccine candidate in later studies and was found to elicit an immune response that was protective against disease in an animal model. This patented technology is currently being investigated for use in patients. As a result of my graduate studies, I have a strong background in microbiology, protein biology, and immunology.
As a postdoctoral researcher, my studies have focused on the intercellular crosstalk between the cystic epithelium and kidney resident macrophages. Using single cell RNA sequencing, I identified two possible clusters of cystic epithelium that shared expression of several genes, including Spp1. My spatial transcriptomics and RNA scope data confirm that Spp1 expression is strongly enriched in cystic epithelium. Further, loss of Spp1 worsened cystic disease suggesting that Spp1 restricts cyst progression, possibly through influencing macrophage accumulation and activation. The results of these studies are currently being assembled into a manuscript. My current project is focused on understanding how macrophages influence the progression of cystic kidney disease. In particular, I found that a specific subset of macrophages, termed Trem2+ cyst associated macrophages (CAM), was significantly enriched in mice with cystic kidney disease compared to non-cystic littermate controls. To test the functional importance of these cells, I have crossed cystic mice to mice lacking Trem2. Preliminary data from these studies indicate that loss of Trem2 worsens cystic kidney disease, suggesting that Trem2+ CAM may restrict cyst progression. In these studies, I will challenge the paradigm that all kidney macrophages promote cyst progression, and instead, pursue the concept that there are beneficial populations of macrophages that can restrict cyst growth.
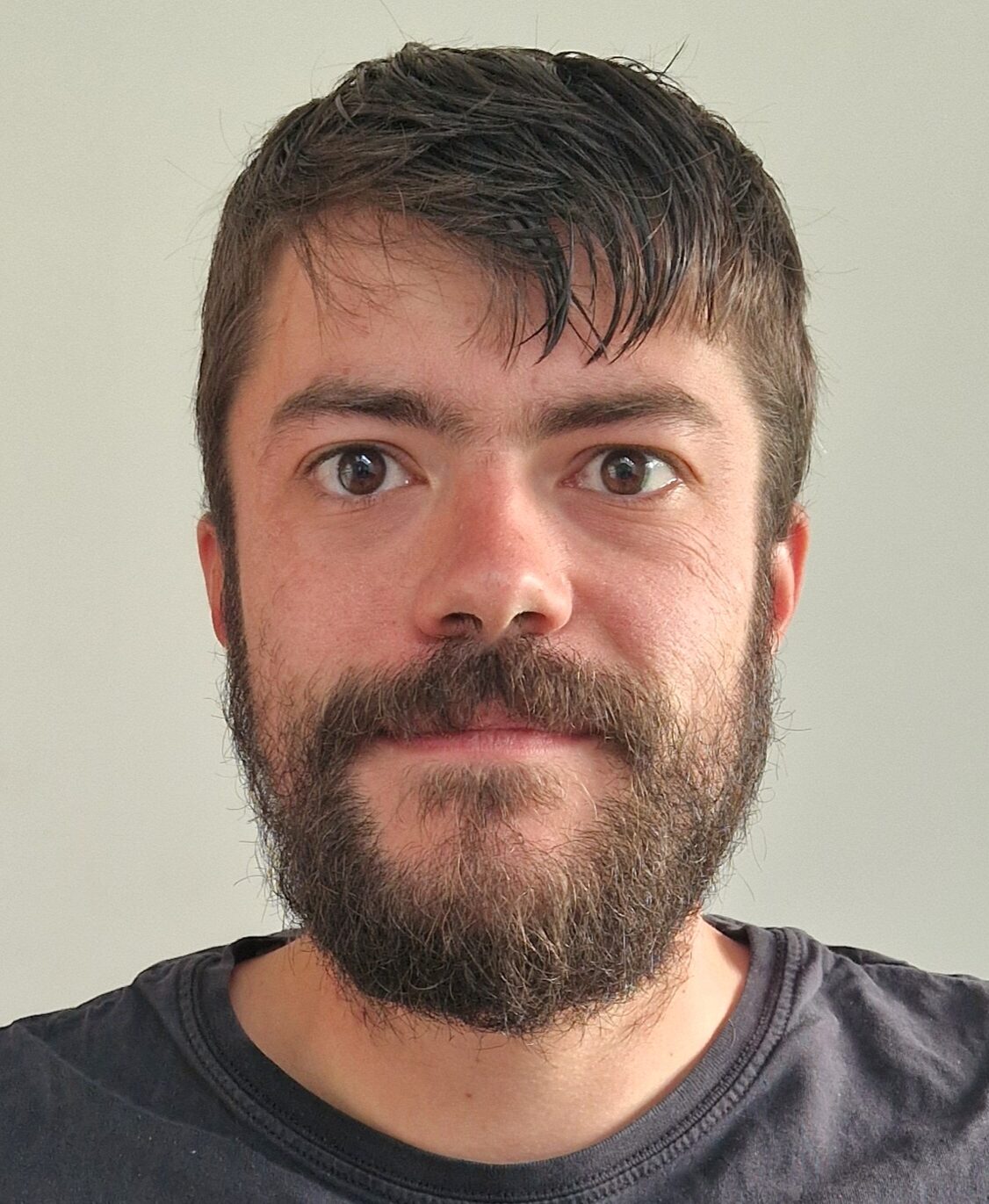
Thomas Naert, Ph.D.
Ghent University
Project Summary
Morphological and molecular insights into vascular complications of ADPKD
A common non-renal manifestation of autosomal dominant polycystic kidney disease (ADPKD) is the development of aneurysms, abnormal outpouchings in the walls of a blood vessels. Unfortunately, aneurysms can rupture leading to life-threatening internal bleeding. Especially when located in the brain vasculature (intracranial aneurysms), rupture is a catastrophic event with a high mortality rate or leading to permanent neurological impairment. These severe vascular outcomes of ADPKD also cluster in certain families. While better quality of care for the renal manifestations of ADPKD carefully becomes available to patients, it is becoming apparent that the cardiovascular complications are still poorly characterized and there is no consensus yet on the clinical management.
Meanwhile, it is becoming accepted that these vascular complications are not simply secondary to kidney pathology. Indeed, alterations in polycystin-1 or -2 expression (PC-1 or 2, protein products of PKD1 or PKD2) directly affect non-renal cell types.
Recently, I used the aquatic model organism Xenopus tropicalis to establish novel animal models for ADPKD, by using CRISPR/Cas9 to target and inactivate either the pkd1 or the pkd2 gene. I can inject this CRISPR/Cas9 molecular scissors into freshly fertilized frog embryos and watch them develop renal cystogenesis in real-time within a time-span of two days (Naert et al, Development 2021, public news release – https://www.eurekalert.org/news-releases/933875).
While our model is also well-positioned to elucidate further insights into the renal manifestations of ADPKD, I here propose to use our unique externally developing animal model to investigate the cardiovascular complications of ADPKD. I will use so-called clearing technologies, which render biological tissues “see-through” and then employ advanced microscopy (light-sheet microscopy) to gather three-dimensional recordings of the entire vasculature in our intact frog tadpoles. Next, I will use state-of-the-art artificial intelligence (deep learning) image processing to automatically process these very large datasets and score for vascular pathology. As such, I intend to use our xenopus ADPKD models to scrutinize the entire cardiovascular structures of Xenopus embryos and investigate morphologically the presence, location and characteristics of intra- and extracranial aneurysms. After locating these, I will use single cell multi-omics (a method to understand how cells are wired) to investigate the abnormal cell signalling leading to vascular disease (finding out which wires are in the wrong position).
I believe that gaining deeper insights into the morphology as well as the signaling programs of cells composing ADPKD-associated aneurysms will yield novel insights, which could then be used to derive novel treatment strategies.
Biography
Thomas Naert, Ph.D is currently a junior postdoctoral research fellow in the laboratory of Dr. Soeren Lienkamp, in the Department of Anatomy at Zurich University. Dr. Naert will soon move as senior postdoctoral fellow in the laboratory of Dr. Kris Vleminckx, in the Department of Biomedical Molecular Biology at Ghent University. Dr. Naert has a longstanding research interest in modeling rare genetic diseases and elucidating their molecular mechanisms using the amphibian model organism Xenopus. His work has provided novel insights in both the molecular drivers of rare cancers (desmoid tumors, glioblastoma, …) as well as rare genetic diseases (ADPKD, renal agenesis, …). Dr. Naert has recently established Xenopus animal models for ADPKD, which are amenable for higher-throughput investigation via employing artificial intelligence and computer vision approaches. These new tools will be used to investigate the cardiovascular manifestations of ADPKD, with an emphasis on intracranial cerebral aneurysms.
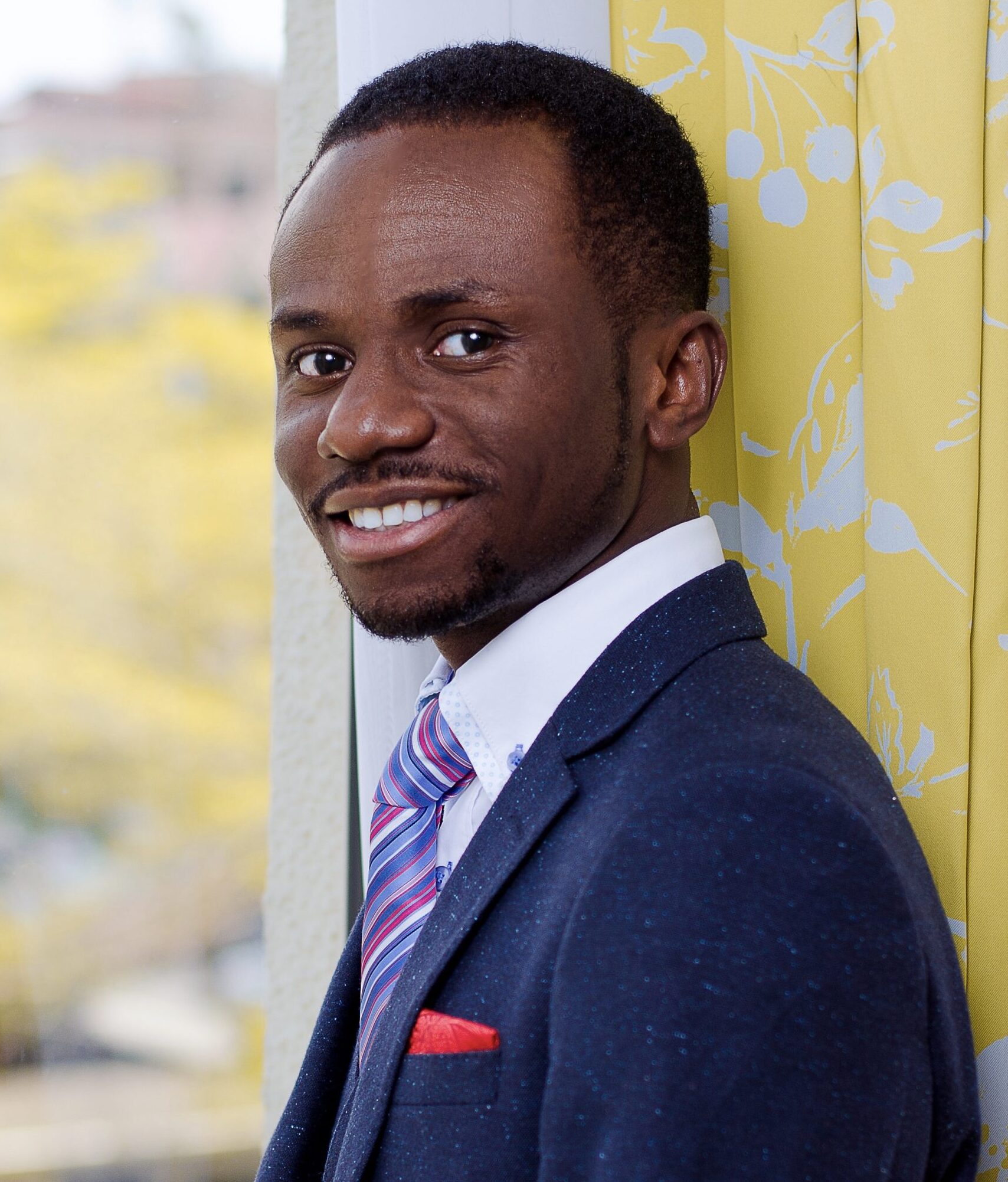
Duuamene Nyimanu, Ph.D.
University of Kansas Medical Center
Project Summary
The Role of the Apelin system in polycystic kidney disease (ADPKD)
Autosomal dominant polycystic kidney disease (ADPKD) is one of the most common and potentially life-threatening diseases. A key feature of ADPKD is the presence of numerous fluid-filled cysts in the kidneys. The size of the cysts increases with time, making the kidneys extremely large and causing them not to function properly. Large kidneys can be very painful and impact the quality of life. ADPKD affects both children and adults, and over 50% of people with the disease develop kidney failure by age 50. Dialysis is the only option for these individuals while waiting for a kidney transplant. Heart disease is one of the most common causes of death in people with ADPKD. Despite FDA approval of tolvaptan, there is still an unmet need for more effective drugs for treating ADPKD due to the unfavorable side effects of tolvaptan. The apelin pathway has emerged as a new drug target that could help individuals with ADPKD. Activation of the apelin pathway improves heart and kidney function and lowers blood pressure in animal models. This study proposes that activating the apelin pathway in PKD will slow cyst growth and improve kidney function. Apelin signaling may also reduce the development of high blood pressure in ADPKD patients. To test these hypotheses, we will activate the apelin pathway in animal models of PKD and determine if this approach slows cyst development and reduces disease progression. We will also determine whether it prevents the activation of pathways responsible for high blood pressure in ADPKD. We will measure apelin levels in the blood of people with ADPKD and normal volunteers to determine if apelin levels can be used to predict disease progression. Apelin has already been tested in humans and found to be safe. Therefore, this study could lead to the discovery of a new treatment approach that could improve the quality of life of people with ADPKD.
Biography
Dr Nyimanu received his Ph.D. in Cardiovascular Pharmacology at the University of Cambridge, England. His doctoral thesis research focused on the role of the apelin pathway in the cardiorenal system. He holds a bachelor’s degree in Biomedicine and a master’s degree in Molecular Medicine from the University of East Anglia, Norwich, England. He also holds a Master of Research (MRes) degree in Medical Sciences (Metabolic and Cardiovascular Disease) from the University of Cambridge, England. He moved to the Jared Grantham Kidney Institute at the University of Kansas Medical Center, Kansas City, Kansas, in 2022 to pursue postdoctoral research training under the mentorship of Dr. Alan Yu.
Core Lab Grant Award
The PKD Foundation’s Core Lab grant program is designed to support research facilities, databases, and services to benefit the entire PKD research community.
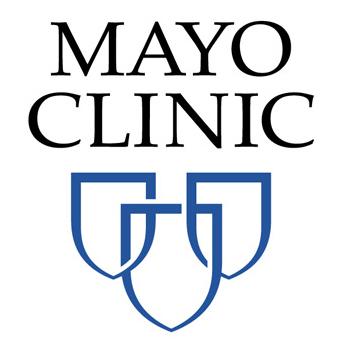
Mayo ADPKD Mutation Database
Mayo Clinic
Project Summary
Mayo ADPKD Mutation Database
In other genetic diseases, such as cystic fibrosis, all patients are routinely molecularly screened so that families know their disease causing mutations. At present this does not happen for ADPKD, partly because the value of the data for diagnostics and prognostics is not generally recognized, and because molecular testing is specialized and expensive due to the complex genomic situation of the PKD1 gene. However, as specialized next generation sequencing panels for ADPKD become available and there is more competition to offer testing, the price of testing is likely to decrease. In addition, the value of the patient knowing their specific mutation is likely to be more widely appreciated as the prognostic value of this data is realized, and genetic data is increasing used to select patients for treatment and clinical trials. In cystic fibrosis, therapies geared to specific mutations are being developed. ADPKD is more complex because of the high level of family specific mutations, but mutation focused treatment strategies are still likely to be tested in this disorder in the next few years. For instance, chaperone treatment may be of value for some missense substitutions and read-through drugs help patients with nonsense mutations, while therapies to overcome splicing defects have also been proposed. Hence, the database will play an increasingly important role as a repository of the accumulating genetic data (paired with clinical and in vitro data), allowing the penetrance of specific mutations to be better determined. In addition, it will function as a source of information about patients that (through their nephrologist) can be selected for treatments and specific clinical trials, including targeting specific mutation groups.
Biography
Visit Mayo ADPKD Mutation Database website